Advancements and Applications of Conductive Polymers: A Review
Emphasizing their types, production techniques, and uses in electronics and energy storage, this paper investigates conductive polymers highlights challenges and future possibilities for technological developments.
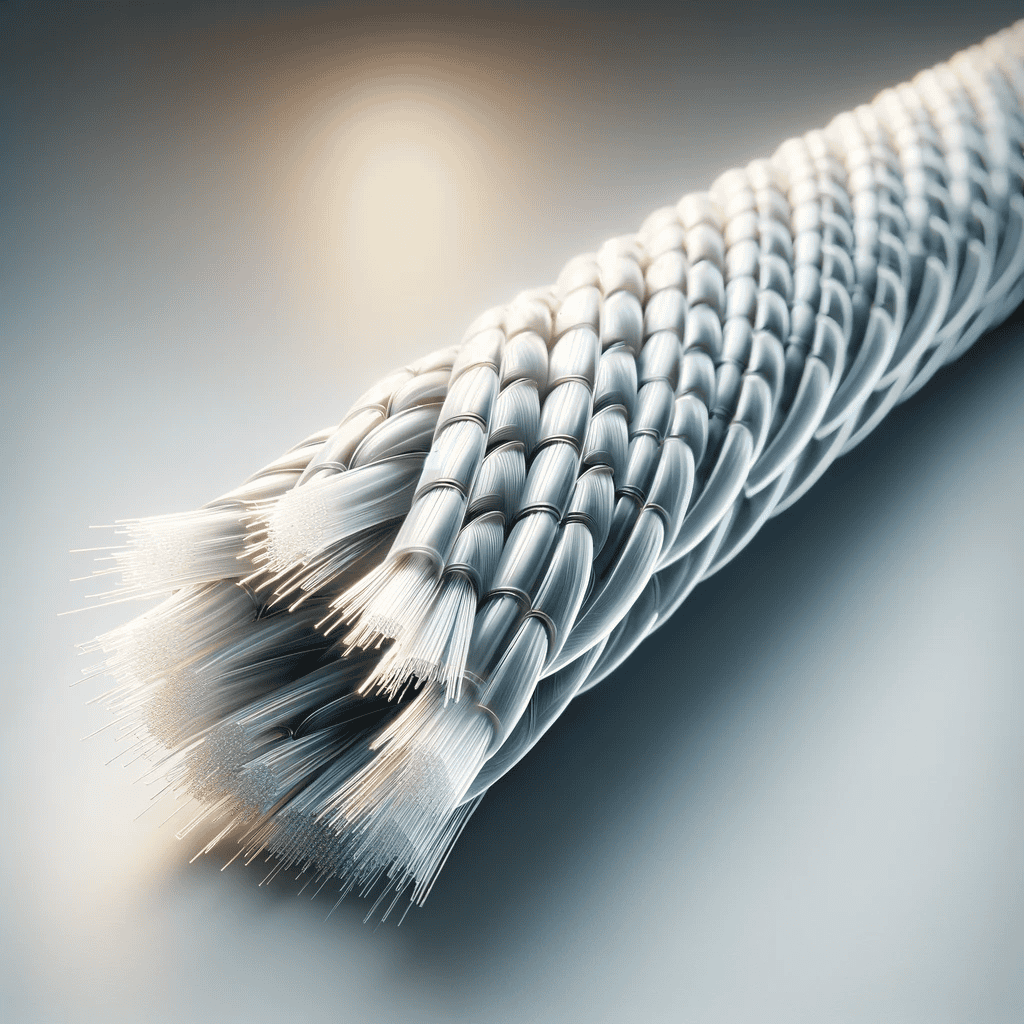
Introduction to Conductive Polymers
A class of materials known as conductive polymers combines the conductivity of organic conductors with the properties of conventional polymers. Because of their special qualities—such as intrinsic conductive networks, adjustable mechanical properties, and easiness of processing—these polymers have attracted great interest in material research (Guo et al., 2023). Doping engineering has driven developments in doping engineering that have defined the historical evolution of conductive polymers and produced materials with great electrical conductivity, such as poly(3,4-ethylenedioxythiophene) (PEDOT). Adding different dopants, such anionic surfactants, into the polymer matrix can help to increase the conductivity of these polymers even more (Fan et al., 2008).
Conductive polymers are important because of their wide spectrum of uses, which include smart electronics, energy conversion/storage, gas sensors, and physical sensors for healthcare monitoring, Tu et al., 2021;, Wang et al., 2012. These polymers have been used in the creation of creative materials such core-shell nanofibers for highly sensitive gas sensors, in which polymeric adsorbents are combined inside conducting polymeric nanostructures (Wang et al., 2012). By providing a mix between conductivity and mechanical strength (Kwon et al., 2011;, Gómez et al., 2021), conductive polymer composites—especially those including carbon nanotubes—have shown promise in many uses, including organic electronics and coatings.
By means of conductive fillers—such as carbon black or inorganic fillers—into polymer matrices, it has been demonstrated that the electrical conductivity of composite materials is improved, so rendering them appropriate for high-performance applications including lithium batteries (“Polymer Composites in Energy Based on Fluid Mechanics”, 2022;, Huy et al., 2021). Furthermore, the regulated inclusion of conductive polymers such as polypyrrole has been demonstrated to lower spark sensitivity in nanothermites, hence underscoring the several uses for these materials (Goetz et al., 2022;, Goetz & Gibot, 2023).
A flexible class of materials with a long history of research and many uses in many different fields, conductive polymers are These polymers remain very important in the progress of material science and technology thanks to developments in doping engineering, composite formulations, and the integration of new additives.
Types and Synthesis of Conductive Polymers
Among the most often used materials in conductive polymers are polyaniline, polypyrrole, and polythiophene. By chemical doping methods with dopants such as dodecyl benzene sulfonic acid (DBSA) Poddar & Patel (2021), polyaniline (PANI) can get high conductivity. Usually including oxidants like ammonium persulfate and ferric chloride (Jagadeshvaran & Bose, 2023), polypyrrole synthesis One further well-known conductive polymer is polythiophene.
Different techniques allow one to synthesize these conductive polymers. Commonly used chemical polymerization methods include oxidative polymerization of moners such aniline, pyrrole, or thiophene (Wang et al., 2004;, Rahy et al., 2008;, Deshmukh et al., 2014). Precise control over the polymerization process and its use to synthesis conductive polymer nanotube structures (Xiao et al., 2007;, Cho et al., 2005;, Cho & Lee, 2008) come from electrochemical methods including electropolymerization. Polypyrrole and poly(3,4-ethylenedioxythiophene) (PEDOT) (Choose et al., 2005) have controlled electrochemical production of conductive polymer nanotubes shown.
Sonoelectrochemical methods have been used in the production of conductive polymers to generate films with particular morphological and adhesion characteristics (Dejeu et al., 2010). Additionally under investigation is the application of surfactants and oxidizing agents to customize the characteristics of resultant conductive polymer nanocomposites (Castillo-Reyes et al., 2014; Arjomandi & Tadayyonfar, 2013). Successful single-step synthesis of hybrid nanotubes, such polypyrrole-gold composites, shows the possibility to generate new composite materials (Sarma et al., 2012).
Key conductive polymers with special qualities that find use in many different disciplines are polyaniline, polypyrrole, and polythiophene. These polymers are synthesized by chemical polymerization using electrochemical methods, therefore enabling exact control over their structure and properties. Novel approaches help to progress conductive polymer synthesis and enable the creation of novel materials with specific uses.
Applications and Future Perspectives
Conductive polymers include polyaniline, polypyrrole, and polythiophene find use in electronics, energy storage, and sensors among other areas. Because they can move between semiconducting and conducting states, these polymers have showed promise in electronics in the development of optoelectronic devices, hence providing flexibility in device design and fabrication Kaloni et al. (2017). Additionally used in energy storage applications are conductive polymers; polythiophene shows promise for usage in charge storage devices and high-frequency applications (Moosvi & Majid, 2017). Conducting polymers’ material flexibilities and tuned conductivities appeal to energy storage technologies (Moosvi & Majid, 2017).
Within the field of sensors, polyaniline and other conductable polymers have been investigated extensively for their chemiresistive sensing properties, therefore allowing the identification of many chemical and biological species (Song & Choi, 2013). Because of their environmental stability and simplicity of synthesis (Virji et al., 2004), polyaniline nanofibers have especially attracted interest for use in gas sensing. These materials have been combined into chemiresistive sensors for gas detection including hydrogen sulfide and ammonia, therefore highlighting its possibilities in environmental and industrial monitoring (Shirsat et al., 2009;, Sadek et al., 2007).
Future advancements in the field of conductive polymers are probably going to concentrate on raising the sensitivity and selectivity of sensors, so improving the performance of energy storage devices, and so broadening the uses of these materials in new technologies. Optimizing the synthesis techniques to guarantee repeatability and scalability, addressing the stability and durability of the materials in real-world conditions, and lowering production costs to enable broad adoption in many sectors define the challenges confronting the commercial scalability of conductive polymers.
Ultimately, conductive polymers have great promise in sensor uses, energy storage, and electronics. Overcoming current difficulties and fully utilizing these materials for next technological developments depend on ongoing research and development projects.
References
(2022). Polymer composites in energy based on fluid mechanics. Academic Journal of Energy, 3(4). https://doi.org/10.38007/re.2022.030406
Fan, B., Mei, X., & Ouyang, J. (2008). Significant conductivity enhancement of conductive poly(3,4-ethylenedioxythiophene):poly(styrenesulfonate) films by adding anionic surfactants into polymer solution. Macromolecules, 41(16), 5971-5973. https://doi.org/10.1021/ma8012459
Goetz, V. and Gibot, P. (2023). Al/sno2 nanothermite esd desensitization by means of the elaboration of tailored sno2–polypyrrole composites. Acs Applied Materials & Interfaces, 15(7), 9830-9840. https://doi.org/10.1021/acsami.2c20700
Goetz, V., Gibot, P., & Spitzer, D. (2022). Spark sensitivity and light signature mitigation of an al/sno2 nanothermite by the controlled addition of a conductive polymer. Chemical Engineering Journal, 427, 131611. https://doi.org/10.1016/j.cej.2021.131611
Guo, X., Sun, Y., Sun, X., Wu, J., Shi, Y., & Pan, L. (2023). Doping engineering of conductive polymers and their application in physical sensors for healthcare monitoring. Macromolecular Rapid Communications, 45(1). https://doi.org/10.1002/marc.202300246
Gómez, I., Sulleiro, M., Mantione, D., & Alegret, N. (2021). Carbon nanomaterials embedded in conductive polymers: a state of the art. Polymers, 13(5), 745. https://doi.org/10.3390/polym13050745
Huy, V., So, S., & Hur, J. (2021). Inorganic fillers in composite gel polymer electrolytes for high-performance lithium and non-lithium polymer batteries. Nanomaterials, 11(3), 614. https://doi.org/10.3390/nano11030614
Khan, Z., Bubnova, O., Jafari, M., Brooke, R., Liu, X., Gabrielsson, R., … & Crispin, X. (2015). Acido-basic control of the thermoelectric properties of poly(3,4-ethylenedioxythiophene)tosylate (pedot-tos) thin films. Journal of Materials Chemistry C, 3(40), 10616-10623. https://doi.org/10.1039/c5tc01952d
Kwon, T., Kim, T., Ali, F., Kang, D., Yoo, M., Bang, J., … & Kim, B. (2011). Size-controlled polymer-coated nanoparticles as efficient compatibilizers for polymer blends. Macromolecules, 44(24), 9852-9862. https://doi.org/10.1021/ma2020134
Tu, Z., Ma, Z., Liang, J., Sheng, L., Shi, Y., & Pan, L. (2021). Prospective on doping engineering of conductive polymers for enhanced interfacial properties. Applied Physics Letters, 119(15). https://doi.org/10.1063/5.0062125
Wang, W., Li, Z., Jiang, T., Zhao, Z., Li, Y., Wang, Z., … & Wang, C. (2012). Sulfonated poly(ether ether ketone)/polypyrrole core–shell nanofibers: a novel polymeric adsorbent/conducting polymer nanostructures for ultrasensitive gas sensors. Acs Applied Materials & Interfaces, 4(11), 6080-6084. https://doi.org/10.1021/am301712t
Arjomandi, J. and Tadayyonfar, S. (2013). Electrochemical synthesis and in situ spectroelectrochemistry of conducting polymer nanocomposites. i. polyaniline/tio2, polyaniline/zno, and polyaniline/tio2+zno. Polymer Composites, 35(2), 351-363. https://doi.org/10.1002/pc.22668
Castillo-Reyes, B., Ovando-Medina, V., González-Ortega, O., Alonso-Dávila, P., Juárez-Ramírez, I., Martínez-Gutiérrez, H., … & Márquez-Herrera, A. (2014). Tio2/polypyrrole nanocomposites photoactive under visible light synthesized by heterophase polymerization in the presence of different surfactants. Research on Chemical Intermediates, 41(11), 8211-8231. https://doi.org/10.1007/s11164-014-1886-0
Cho, S. and Lee, S. (2008). Fast electrochemistry of conductive polymer nanotubes: synthesis, mechanism, and application. Accounts of Chemical Research, 41(6), 699-707. https://doi.org/10.1021/ar7002094
Cho, S., Choi, D., Kim, S., & Lee, S. (2005). Electrochemical synthesis and fast electrochromics of poly(3,4-ethylenedioxythiophene) nanotubes in flexible substrate. Chemistry of Materials, 17(18), 4564-4566. https://doi.org/10.1021/cm050729c
Dejeu, J., Taouil, A., Rougeot, P., Lakard, S., Lallemand, F., & Lakard, B. (2010). Morphological and adhesive properties of polypyrrole films synthesized by sonoelectrochemical technique. Synthetic Metals, 160(23-24), 2540-2545. https://doi.org/10.1016/j.synthmet.2010.10.002
Deshmukh, P., Pusawale, S., Shinde, N., & Lokhande, C. (2014). Growth of polyaniline nanofibers for supercapacitor applications using successive ionic layer adsorption and reaction (silar) method. Journal of the Korean Physical Society, 65(1), 80-86. https://doi.org/10.3938/jkps.65.80
Jagadeshvaran, P. and Bose, S. (2023). Evolution of surface engineering in the development of textile-based emi shields─a review. Acs Applied Electronic Materials, 5(4), 1947-1969. https://doi.org/10.1021/acsaelm.2c01733
Poddar, A. and Patel, S. (2021). Synthesis, characterization and applications of conductive polymers: a brief review. Polymers for Advanced Technologies, 32(12), 4616-4641. https://doi.org/10.1002/pat.5483
Rahy, A., Sakrout, M., Manohar, S., Cho, S., & Yang, D. (2008). Polyaniline nanofiber synthesis by co-use of ammonium peroxydisulfate and sodium hypochlorite. Chemistry of Materials, 20(15), 4808-4814. https://doi.org/10.1021/cm703678m
Sarma, A., Sanyal, M., Rahman, A., & Satpati, B. (2012). Hybrid nanotubes: single step formation of homogeneous nanotubes of polypyrrole-gold composites and novel switching transition of resistance beyond liquid nitrogen temperature. Journal of Applied Physics, 112(4). https://doi.org/10.1063/1.4746743
Wang, Y., Liu, Z., Han, B., Sun, Z., Zhang, S., & Yang, G. (2004). Facile synthesis of polyaniline nanofibers using chloroaurate acid as the oxidant. Langmuir, 21(3), 833-836. https://doi.org/10.1021/la047442z
Xiao, R., Cho, S., Liu, R., & Lee, S. (2007). Controlled electrochemical synthesis of conductive polymer nanotube structures. Journal of the American Chemical Society, 129(14), 4483-4489. https://doi.org/10.1021/ja068924v
Kaloni, T., Giesbrecht, P., Schreckenbach, G., & Freund, M. (2017). Polythiophene: from fundamental perspectives to applications. Chemistry of Materials, 29(24), 10248-10283. https://doi.org/10.1021/acs.chemmater.7b03035
Moosvi, S. and Majid, K. (2017). Spectroscopic, morphological, thermal and dielectrical analysis of composite of polythiophene with photoactive transition metal complex of w(iv). Bulletin of Materials Science, 40(6), 1151-1158. https://doi.org/10.1007/s12034-017-1472-3
Sadek, A., Włodarski, W., Kalantar‐zadeh, K., Baker, C., & Kaner, R. (2007). Doped and dedoped polyaniline nanofiber based conductometric hydrogen gas sensors. Sensors and Actuators a Physical, 139(1-2), 53-57. https://doi.org/10.1016/j.sna.2006.11.033
Shirsat, M., Bangar, M., Deshusses, M., Myung, N., & Mulchandani, A. (2009). Polyaniline nanowires-gold nanoparticles hybrid network based chemiresistive hydrogen sulfide sensor. Applied Physics Letters, 94(8). https://doi.org/10.1063/1.3070237
Song, E. and Choi, J. (2013). Conducting polyaniline nanowire and its applications in chemiresistive sensing. Nanomaterials, 3(3), 498-523. https://doi.org/10.3390/nano3030498
Virji, S., Huang, J., Kaner, R., & Weiller, B. (2004). Polyaniline nanofiber gas sensors: examination of response mechanisms. Nano Letters, 4(3), 491-496. https://doi.org/10.1021/nl035122e