Carbohydrate Chemistry: Structure, Synthesis, and Innovative Applications
Emphasizing structural variety, sophisticated synthesis methods, and useful applications in medicines, food science, and materials engineering, this study investigates carbohydrate chemistry highlighting future research paths and possible improvements.
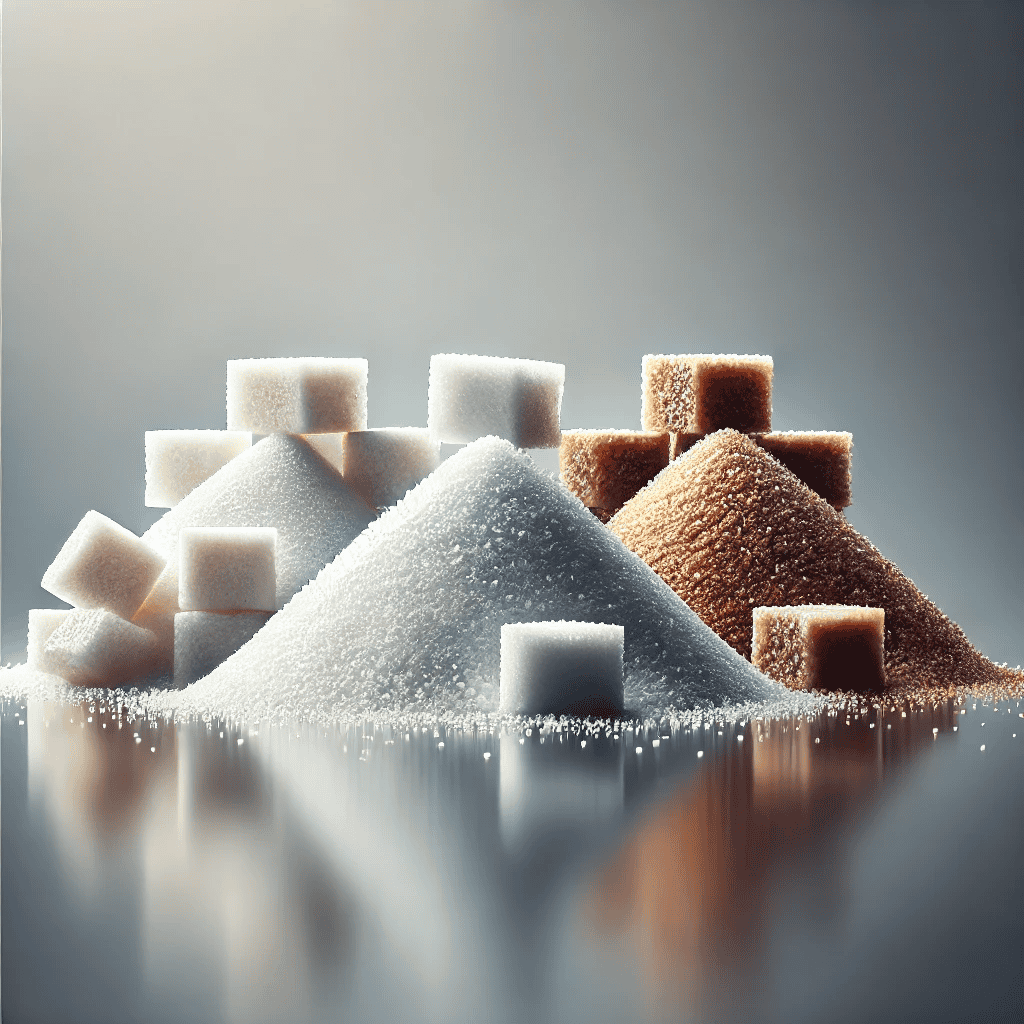
1. Fundamentals of Carbohydrate Structure
Monosaccharides, disaccharides, and polysaccharides are three primary classes of important biomolecules distinguished by structure and composition. Whereas disaccharides comprise two monosaccharide units joined together, monosaccharides are single sugar units. Made comprised of several monosaccharide units joined together by glycosidic connections, polysaccharides are complex carbohydrates (Cummings & Stephen, 2007).
Carbohydrates are categorized according to their degree of polymerization, or DP. Monosaccharides are those with a DP of 1–2; oligosaccharides are those with a DP of 3–9; polysaccharides are those with a DP more than or equal to 10 (Cummings & Stephen, 2007). Usually a hexosamine and a uronic acid, polysaccharides such as glycosaminoglycans (GAGs) are lengthy unbranched polymers made of repeating disaccharide units (Bantzi et al., 2015).
Variations in glycosidic connections and functional groups produce the structural variety of carbohydrates. For example, proteoglycans—which play vital roles in the extracellular matrix—consist of a core protein coupled to glycosaminoglycan chains via O-glycosidic connections. Moreover, the ionic environment and the particular glycosidic connections in the molecule shape carbohydrates (Schefer et al., 2014; Zhang et al., 2017).
With their classification depending on the number of sugar units they contain, carbohydrates show a broad spectrum of structures from simple monosaccharides to complicated polysaccharides. These molecules’ glycosidic connections and functional groups help to explain their structural variety and biological purposes.
2. Chemical Synthesis Techniques
Different chemical techniques are used to synthesis complicated carbohydrates including oligosaccharides and polysaccharides with an eye toward glycosylation schemes and enzyme-catalyzed processes. Because of their complex architectures, chemical synthesis of oligosaccharides calls for careful planning including the choice of glycosylation techniques, synthetic methodologies, and protecting groups (Muthana et al., 2009). Central in synthetic carbohydrate chemistry, glycosylation is absolutely important in the regulated assembly of complex oligosaccharides and glycoconjugates from monosaccharide substrates (Qin & Ye, 2021).
By providing a scalable and effective method to generate well defined and pure carbohydrate-containing compounds, chemical synthesis helps to comprehend their purposes and generates therapeutic medicines (Li et al., 2020). Chemical synthesis still has a great difficulty developing techniques for the construction of lengthy, branching, and complicated glycans with many 1,2-cis glycosidic linkages (Zhang et al., 2021). One prominent approach to simplify the chemical synthesis of oligosaccharides (Zhang et al., 2020) is one-pot glycosylation procedures depending on N-phenyltrifluoroacetimidate (PTFAI) and Yu glycosylation.
Enzyme-catalyzed reactions, such chemoenzymatic synthesis, offer a means for regio- and stereoselective glycosylation of chemically derived substrates by enzymes like glycosyltransferases, glycosidases, and glycosynthases, so enabling the parallel and combinatorial synthesis of natural or non-natural carbohydrates of biological relevance (Hsu et al., 2011). Aiming to improve synthetic efficiency, enzymatic synthesis employing CS biosynthesis enzymes is also under investigation as a different method to manufacture homogenous oligosaccharides.
The chemical synthesis of complicated carbohydrates entails, all things considered, a variety of techniques including glycosylation techniques and enzyme-catalyzed processes. Producing clearly characterized carbohydrate compounds, grasping their purposes, and creating possible medicinal medicines depend on these techniques.
3. Biotechnological and Industrial Applications
Because of their many uses, carbohydrates are indispensable in many fields, including material sciences, food manufacture, and medicine. Recent developments in the biotechnological and industrial uses of carbohydrates have produced creative ideas and great future possibilities.
In the pharmaceutical sector, drug research and delivery systems depend on carbs absolutely. Extensive research has been done on lectins, proteins that identify particular carbohydrate structures, for their biomedical uses including disease biomarkers Coelho et al. (2017) and aberrant cell detection. Furthermore useful in food, cosmetics, and medicine are the synthesis of trehalose, a stable disaccharide (Sinkowicz & Synowiecki, 2009).
Microalgae have attracted interest in food production for their high-value lipids and functional chemicals, which find use in the pharmaceutical and bioenergy sectors (Mutanda et al., 2020). Extraction, degumming of plant fibers, and biomedicine (Trincone, 2018) among other uses in the food sector have also been investigated for marine carbohydrate-hydrolyzing enzymes.
The special characteristics of carbohydrates help the material sciences sector create biomaterials with new purposes. New possibilities for producing supramolecular biomaterials with favorable characteristics for medicinal and biotechnological uses present themselves from self-assembling peptides changed with different carbohydrate chemistries (Restuccia et al., 2019). Furthermore, the functionalizing of glass surfaces via thiol-ene photochemistry emphasizes the need of surface engineering in materials science and biotechnology (Bertin & Schlaad, 2009).
Carbohydrates find usage in bio-based products produced in the industrial sector including animal feed, textiles, and biofuels. Production of bio-based goods depends critically on carbohydrate-active enzymes (CAZymes), whose industrial uses pique great interest for researchers (Han et al., 2021). Moreover, the generation of exopolysaccharides by psychrophilic yeasts shows possible uses in cryoprotective compounds, biofuels, and bioremediation ( Rusinova‐Videva et al., 2022).
Anticipating future developments, the biotechnological uses of carbohydrates are set for even more creativity. From pharmaceuticals to materials research, developments in enzyme technology, microbial bioprocessing, and the creation of new carbohydrate-based materials should propel advancement in all kinds of sectors. Making use of the several features of carbohydrates has great possibility to solve present problems and provide sustainable solutions in many different fields.
References
Bantzi, M., Rigol, S., & Giannis, A. (2015). Synthesis of a hexasaccharide partial sequence of hyaluronan for click chemistry and more. Beilstein Journal of Organic Chemistry, 11, 604-607. https://doi.org/10.3762/bjoc.11.67
Cummings, J. and Stephen, A. (2007). Carbohydrate terminology and classification. European Journal of Clinical Nutrition, 61(S1), S5-S18. https://doi.org/10.1038/sj.ejcn.1602936
Lynch, M., Barallobre-Barreiro, J., Jahangiri, M., & Mayr, M. (2016). Vascular proteomics in metabolic and cardiovascular diseases. Journal of Internal Medicine, 280(4), 325-338. https://doi.org/10.1111/joim.12486
Schefer, L., Adamčík, J., & Mezzenga, R. (2014). Unravelling secondary structure changes on individual anionic polysaccharide chains by atomic force microscopy. Angewandte Chemie, 53(21), 5376-5379. https://doi.org/10.1002/anie.201402855
Zhang, W., Turney, T., Meredith, R., Pan, Q., Sernau, L., Wang, X., … & Serianni, A. (2017). Conformational populations of β-(1→4) o-glycosidic linkages using redundant nmr j-couplings and circular statistics. The Journal of Physical Chemistry B, 121(14), 3042-3058. https://doi.org/10.1021/acs.jpcb.7b02252
Hsu, C., Hung, S., Wu, C., & Wong, C. (2011). Toward automated oligosaccharide synthesis. Angewandte Chemie, 50(50), 11872-11923. https://doi.org/10.1002/anie.201100125
Li, J., Su, G., & Liu, J. (2017). Enzymatic synthesis of homogeneous chondroitin sulfate oligosaccharides. Angewandte Chemie, 56(39), 11784-11787. https://doi.org/10.1002/anie.201705638
Li, P., He, H., Zhang, Y., Yang, R., Xu, L., Chen, Z., … & Xiao, G. (2020). Glycosyl ortho-(1-phenylvinyl)benzoates versatile glycosyl donors for highly efficient synthesis of both o-glycosides and nucleosides. Nature Communications, 11(1). https://doi.org/10.1038/s41467-020-14295-z
Muthana, S., Cao, H., & Chen, X. (2009). Recent progress in chemical and chemoenzymatic synthesis of carbohydrates. Current Opinion in Chemical Biology, 13(5-6), 573-581. https://doi.org/10.1016/j.cbpa.2009.09.013
Qin, X. and Ye, X. (2021). Donor preactivation‐based glycosylation: an efficient strategy for glycan synthesis. Chinese Journal of Chemistry, 39(3), 531-542. https://doi.org/10.1002/cjoc.202000484
Zhang, Y., Chen, Z., Huang, Y., He, S., Yang, X., Wu, Z., … & Xiao, G. (2020). Modular synthesis of nona‐decasaccharide motif from psidium guajava polysaccharides: orthogonal one‐pot glycosylation strategy. Angewandte Chemie, 59(19), 7576-7584. https://doi.org/10.1002/anie.202000992
Zhang, Y., He, H., Chen, Z., Huang, Y., Xiang, G., Li, P., … & Xiao, G. (2021). Merging reagent modulation and remote anchimeric assistance for glycosylation: highly stereoselective synthesis of α‐glycans up to a 30‐mer. Angewandte Chemie, 60(22), 12597-12606. https://doi.org/10.1002/anie.202103826
Bertin, A. and Schlaad, H. (2009). Mild and versatile (bio-)functionalization of glass surfaces via thiol−ene photochemistry. Chemistry of Materials, 21(24), 5698-5700. https://doi.org/10.1021/cm902176n
Coelho, L., Silva, P., Lima, V., Pontual, E., Napoleão, T., & Correia, M. (2017). Lectins, interconnecting proteins with biotechnological/pharmacological and therapeutic applications. Evidence-Based Complementary and Alternative Medicine, 2017, 1-22. https://doi.org/10.1155/2017/1594074
Han, S., Kim, B., Jang, J., Park, H., & Oh, T. (2021). Complete genome sequence of arthrobacter sp. pamc25564 and its comparative genome analysis for elucidating the role of cazymes in cold adaptation. BMC Genomics, 22(1). https://doi.org/10.1186/s12864-021-07734-8
Mutanda, T., Naidoo, D., Bwapwa, J., & Anandraj, A. (2020). Biotechnological applications of microalgal oleaginous compounds: current trends on microalgal bioprocessing of products. Frontiers in Energy Research, 8. https://doi.org/10.3389/fenrg.2020.598803
Restuccia, A., Seroski, D., Kelley, K., O’Bryan, C., Kurian, J., Knox, K., … & Hudalla, G. (2019). Hierarchical self-assembly and emergent function of densely glycosylated peptide nanofibers. Communications Chemistry, 2(1). https://doi.org/10.1038/s42004-019-0154-z
Rusinova‐Videva, S., Ognyanov, M., Georgiev, Y., Kambourova, M., Adamov, A., & Krasteva, V. (2022). Production and chemical characterization of exopolysaccharides by antarctic yeasts vishniacozyma victoriae and tremellomycetes sp.. Applied Sciences, 12(4), 1805. https://doi.org/10.3390/app12041805
Sinkiewicz, I. and Synowiecki, J. (2009). Activity and primary characterization of enzyme fromthermus rubercells catalyzing conversion of maltose into trehalose. Journal of Food Biochemistry, 33(1), 122-133. https://doi.org/10.1111/j.1745-4514.2008.00208.x
Trincone, A. (2018). Update on marine carbohydrate hydrolyzing enzymes: biotechnological applications. Molecules, 23(4), 901. https://doi.org/10.3390/molecules23040901