Advances in Microdroplet Chemistry: Catalysts to Applications
The transforming effect of microdroplet chemistry on reaction efficiency is investigated in this review together with synthesis and applications throughout medicines and catalysis as well as future possibilities and obstacles.
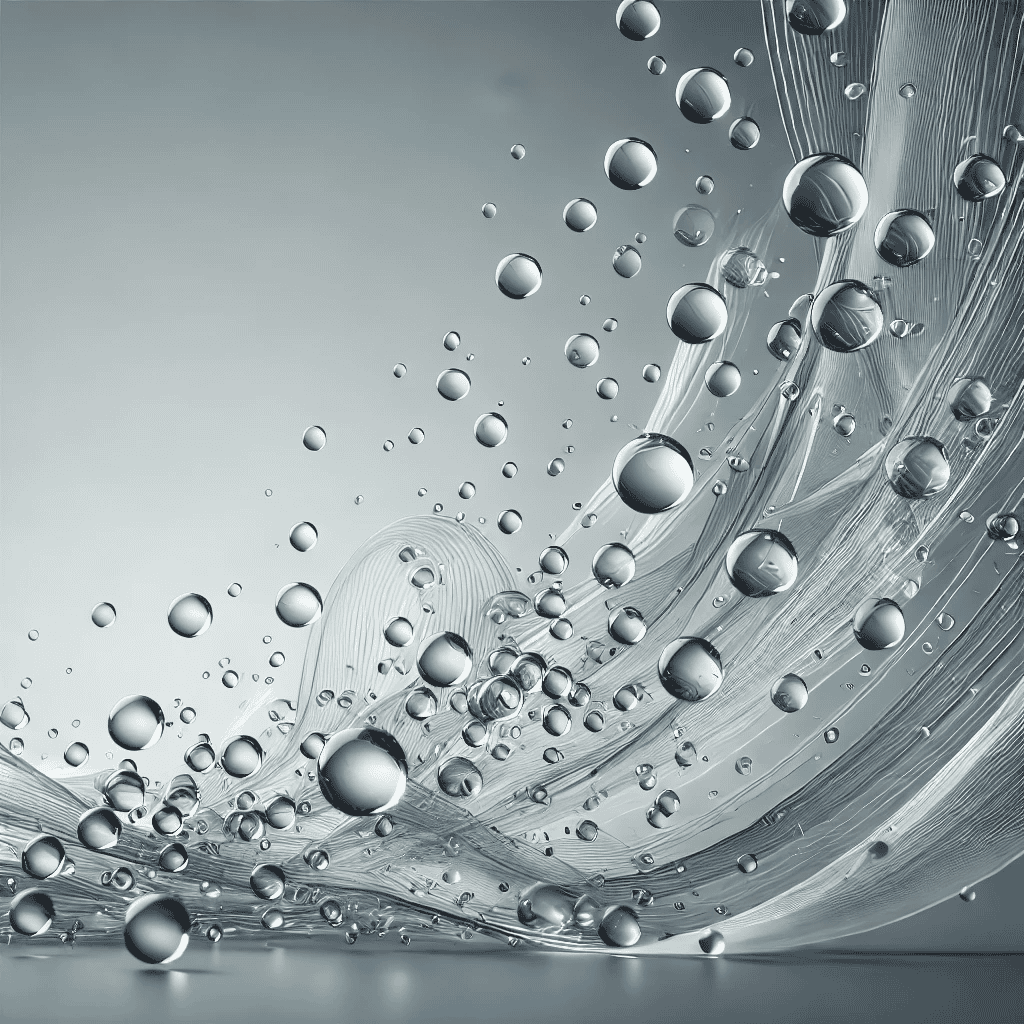
Introduction to Microdroplet Chemistry
Emerging as a major platform for performing reactions, microdroplets provide special kinetic and thermodynamic characteristics not usually found in bulk solutions (Xiong et al., 2020). Accelerated kinetics seen in microdroplets show increases ranging from 2 to 6 orders of magnitude when compared to bulk solutions (Qiu, 2024). Microdroplets’ unique interfacial chemistry is responsible for this acceleration; quicker reactions than in bulk systems follow from this (Huang et al., 2021). High electric fields found in water microdroplets have been demonstrated to catalyze fast and spontaneous reactions, hence greatly speeding chemical reactions (Zhu, 2023).
Furthermore, microdroplet chemistry has been shown to enable several reactions, including hydration of alkynes, with ultrahigh acceleration, complete conversion, and moderate reaction conditions, thus appealing for sustainable and green chemical methods (Zheng et al., 2021). Without catalysts, the special environment of microdroplets has allowed chemoselective reactions—such as the N-alkylation of indoles—to occur (Gnanamani et al., 2020). Furthermore proven to cause spontaneous reduction reactions are microdroplets, which provide a basis for green chemistry by electrochemically activating water molecules without outside reducing chemicals (Lee et al., 2019).
Moreover, microdroplets have been used to speed several reactions, including SuFEx reactions and aza-Michael addition, thereby highlighting the general use of this technique in advancing reaction kinetics (Ghosh et al., 2022). Microdroplets have also made abiotic synthesis of several molecules possible, including uridine ribonucleoside and sugar phosphates possible, therefore underscoring the potential of microdroplet chemistry in enabling chemical reactions and molecular organization (Nam et al., 2017).
All things considered, microdroplet chemistry is a novel discipline with fast reaction kinetics, unusual reaction paths, and possibility for green and sustainable chemical methods. Using the unique characteristics of microdroplets allows scientists to investigate fresh reaction pathways and improve the effectiveness of chemical procedures.
Applications of Microdroplets in Chemistry
Because of its special qualities and ability, microdroplets have been extensively applied in several disciplines including synthetic chemistry, enzyme catalysis, and pharmacological research. Microdroplets have been efficiently applied in synthetic chemistry to hasten reactions in confined conditions, hence improving reaction rates and yields relative to bulk phase processes “undefined” (2018). Green chemistry techniques have been supported and reaction acceleration accelerated by the concentration augmentation of molecules at the microdroplet interface (Xue, 2023; Xiong et al., 2020).
Microdroplets have been used in enzyme catalysis to accelerate enzymatic reactions, hence improving processes including digestion and protein sequencing (Zhong et al., 2020). Providing a platform for quick and efficient protein analysis (Zhong et al., 2020), aqueous microdroplets have especially helped to increase the efficiency of enzymatic processes. Microdroplets mass spectrometry has also been very important in accelerating processes and extending mass spectrometry technique use (Kafeenah et al., 2021).
Moreover, microdroplets have proved indispensable in the synthesis of pharmaceutical compounds, allowing water microdroplets (Meng et al., 2023) one-step synthesis of molecules including phenylacetic acid. Pharmaceutical synthesis has underlined the ability of microdroplets to enable reactions without the necessity of catalysts, thereby showing the possibility for simplified and quick drug development processes (Meng et al., 2023).
Microdroplets have also been essential in many uses, including biphasic extraction for ultra-trace analysis, microreactors for fast compound synthesis, and reactive laser ablation electrospray ionization for click reaction (Zhang et al., 2022; Zhang et al., 2018; Geenen et al., 2018). Microdroplets’ fast reaction kinetics have driven developments in mass spectrometry, catalysis, and peptide synthesis, therefore proving the broad influence of microdroplet technology across several sectors (Wei et al., 2020; Wang et al., 2021).
Ultimately by offering a platform for speedier reactions, better enzymatic processes, and effective pharmaceutical compound synthesis, microdroplets have greatly affected the domains of chemistry, enzyme catalysis, and pharmaceutical research. The unique qualities of microdroplets have opened the path for creative ideas and applications, therefore advancing several fields of science.
Future Perspectives and Challenges
Microdroplet technology promises interesting directions for developments in many other disciplines in future studies. Development of droplet microarrays, which provide high-throughput applications and surface patterning capabilities Feng et al. (2018) is one main area of research. By allowing effective and parallelized experimentation, these microarrays could transform research and cause major developments in disciplines such microfluidics, biology, and genetics.
The full potential of microdroplet systems is hampered by automation of routing microdroplets and iterative processes within them still presents a difficulty (Dolega et al., 2012). Overcoming this challenge might result in improved efficiency and scalability in microdroplets-based systems, therefore opening the path for more simplified and automated procedures in industrial and research contexts.
In ultra-high throughput testing and replicating circumstances similar to single cells, microfluidic systems producing sub-nanoliter droplets scattered within an immiscible continuous phase have showed promise (Huebner et al., 2008). Expanding on this capacity could provide fresh opportunities for compartmentalizing biological and chemical interactions, provide understanding of cellular processes, and provide new directions of research.
Uncontrolled crystallization continues to be a challenge in the scalable manufacturing of arrays of perovskite single crystals with regulated form and location (Gu et al., 2023). Dealing with this difficulty could result in developments in materials science as well as in the creation of integrated devices with customized features for different uses.
Technical difficulties in capillary-based microfluidic device manufacture hinder repeatability and scalability (Zhang et al., 2022). By improving the dependability and scalability of microfluidic devices, overcoming these obstacles could open more general uses in materials research, chromatography, and extraction.
Finally, future studies in microdroplets should concentrate on solving issues with regulated manufacturing techniques, scalability, and automation. Microdroplets systems can fully realize their possibilities in transforming several scientific and industrial uses by addressing these challenges.
References
Ghosh, J., Mendoza, J., & Cooks, R. (2022). Accelerated and concerted aza‐michael addition and sufex reaction in microdroplets in unitary and high‐throughput formats. Angewandte Chemie, 61(50). https://doi.org/10.1002/anie.202214090
Gnanamani, E., Yan, X., & Zare, R. (2020). Chemoselective n‐alkylation of indoles in aqueous microdroplets. Angewandte Chemie, 132(8), 3093-3096. https://doi.org/10.1002/ange.201913069
Huang, K., Wei, Z., & Cooks, R. (2021). Accelerated reactions of amines with carbon dioxide driven by superacid at the microdroplet interface. Chemical Science, 12(6), 2242-2250. https://doi.org/10.1039/d0sc05625a
Lee, J., Samanta, D., Nam, H., & Zare, R. (2019). Micrometer-sized water droplets induce spontaneous reduction. Journal of the American Chemical Society, 141(27), 10585-10589. https://doi.org/10.1021/jacs.9b03227
Nam, I., Lee, J., Nam, H., & Zare, R. (2017). Abiotic production of sugar phosphates and uridine ribonucleoside in aqueous microdroplets. Proceedings of the National Academy of Sciences, 114(47), 12396-12400. https://doi.org/10.1073/pnas.1714896114
Qiu, L. (2024). Spontaneous oxidation in aqueous microdroplets: water radical cation as primary oxidizing agent. Angewandte Chemie, 136(17). https://doi.org/10.1002/ange.202400118
Xiong, H., Lee, J., Zare, R., & Min, W. (2020). Strong concentration enhancement of molecules at the interface of aqueous microdroplets. The Journal of Physical Chemistry B, 124(44), 9938-9944. https://doi.org/10.1021/acs.jpcb.0c07718
Zheng, B., Jin, X., Liu, J., & Cheng, H. (2021). Accelerated metal-free hydration of alkynes within milliseconds in microdroplets. Acs Sustainable Chemistry & Engineering, 9(12), 4383-4390. https://doi.org/10.1021/acssuschemeng.1c00887
Zhu, C. (2023). High electric fields on water microdroplets catalyze spontaneous and fast reactions in halogen-bond complexes. Journal of the American Chemical Society, 145(39), 21207-21212. https://doi.org/10.1021/jacs.3c08818
(2018). Untitled. Academic Journal of Polymer Science, 1(1). https://doi.org/10.19080/ajop.01.1
Geenen, F., Franssen, M., Zuilhof, H., & Nielen, M. (2018). Reactive laser ablation electrospray ionization time-resolved mass spectrometry of click reactions. Analytical Chemistry, 90(17), 10409-10416. https://doi.org/10.1021/acs.analchem.8b02290
Kafeenah, H., Jen, H., & Chen, S. (2021). Microdroplet mass spectrometry: accelerating reaction and application. Electrophoresis, 43(1-2), 74-81. https://doi.org/10.1002/elps.202100208
Meng, Y., Gnanamani, E., & Zare, R. (2023). One-step formation of pharmaceuticals having a phenylacetic acid core using water microdroplets. Journal of the American Chemical Society, 145(14), 7724-7728. https://doi.org/10.1021/jacs.3c00773
Wang, W., Qiao, L., He, J., Ju, Y., Yu, K., Kan, G., … & Jiang, J. (2021). Water microdroplets allow spontaneously abiotic production of peptides. The Journal of Physical Chemistry Letters, 12(24), 5774-5780. https://doi.org/10.1021/acs.jpclett.1c01083
Wei, Z., Li, Y., Cooks, R., & Yan, X. (2020). Accelerated reaction kinetics in microdroplets: overview and recent developments. Annual Review of Physical Chemistry, 71(1), 31-51. https://doi.org/10.1146/annurev-physchem-121319-110654
Xiong, H., Lee, J., Zare, R., & Min, W. (2020). Strong concentration enhancement of molecules at the interface of aqueous microdroplets. The Journal of Physical Chemistry B, 124(44), 9938-9944. https://doi.org/10.1021/acs.jpcb.0c07718
Xue, L. (2023). Water microdroplet chemistry for accelerating green thiocyanation and discovering water-controlled divergence. Acs Sustainable Chemistry & Engineering, 11(34), 12780-12789. https://doi.org/10.1021/acssuschemeng.3c03313
Zhang, W., Yang, S., Lin, Q., Cheng, H., & Liu, J. (2018). Microdroplets as microreactors for fast synthesis of ketoximes and amides. The Journal of Organic Chemistry, 84(2), 851-859. https://doi.org/10.1021/acs.joc.8b02669
Zhang, X., Song, Y., Xu, T., & Zhang, X. (2022). Biphasic microdroplet–microdroplet extraction for ultra‐trace enriching analysis. Advanced Materials Interfaces, 9(25). https://doi.org/10.1002/admi.202200817
Zhong, X., Chen, H., & Zare, R. (2020). Ultrafast enzymatic digestion of proteins by microdroplet mass spectrometry. Nature Communications, 11(1). https://doi.org/10.1038/s41467-020-14877-x
Dolega, M., Jakiela, S., Razew, M., Rakszewska, A., Cybulski, O., & Garstecki, P. (2012). Iterative operations on microdroplets and continuous monitoring of processes within them; determination of solubility diagrams of proteins. Lab on a Chip, 12(20), 4022. https://doi.org/10.1039/c2lc40174f
Feng, W., Ueda, E., & Levkin, P. (2018). Droplet microarrays: from surface patterning to high‐throughput applications. Advanced Materials, 30(20). https://doi.org/10.1002/adma.201706111
Gu, Z., Zhao, Y., Zhang, Y., Huang, Z., Zhou, Z., Wang, K., … & Song, Y. (2023). Ion aggregation-induced crystallization strategy for printing morphology-controllable perovskite single-crystal arrays and integrated devices. Chemistry of Materials, 35(13), 5127-5134. https://doi.org/10.1021/acs.chemmater.3c00755
Huebner, A., Sharma, S., Srisa-Art, M., Hollfelder, F., Edel, J., & deMello, A. (2008). Microdroplets: a sea of applications?. Lab on a Chip, 8(8), 1244. https://doi.org/10.1039/b806405a
Zhang, S., Wang, B., Xue, C., & Chen, X. (2022). Fabrication of diverse microparticles in a unified microfluidic configuration. Advanced Materials Technologies, 8(1). https://doi.org/10.1002/admt.202200680