Sensor Innovations Transforming Analytical Chemistry
This review investigates current advances in sensor technology and their transformational impact on analytical chemistry. It emphasizes gains in sensitivity and selectivity, examines obstacles, and forecasts future prospects for study and application.
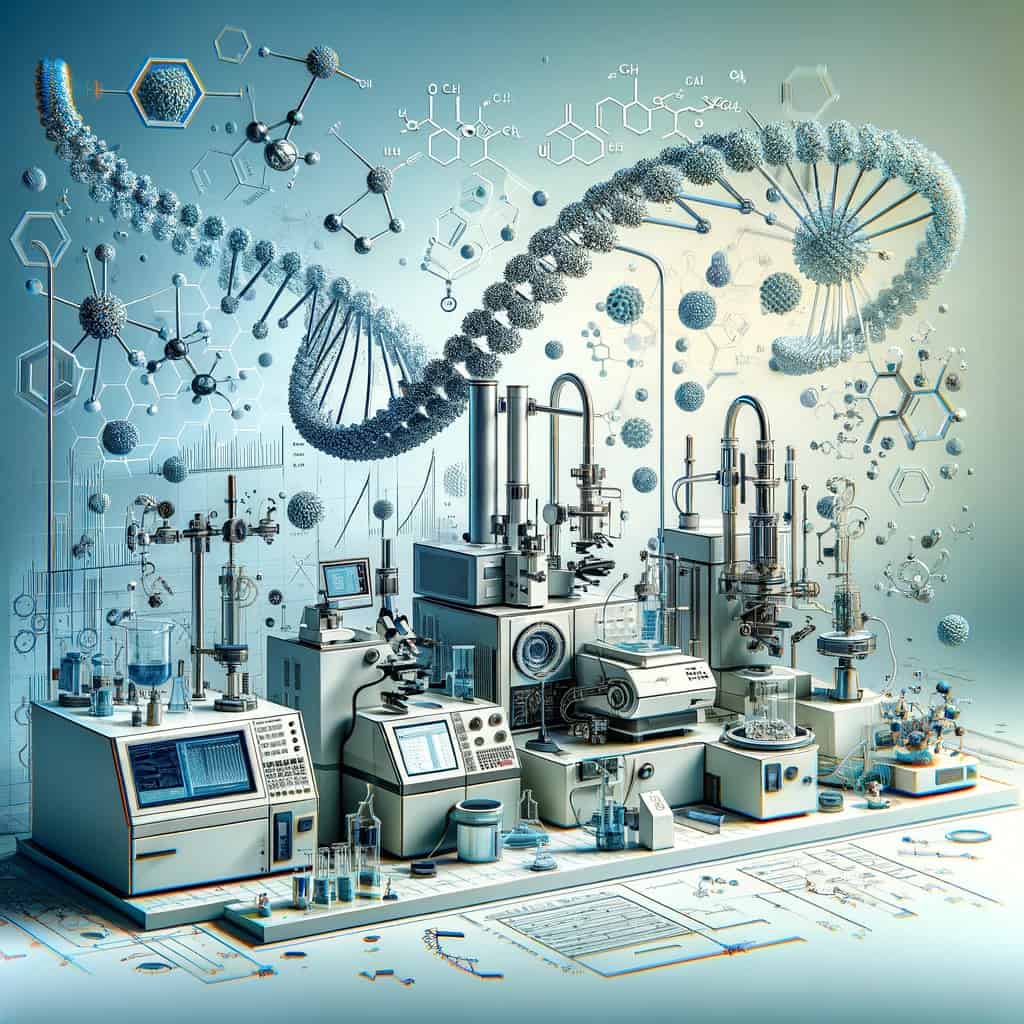
1. Introduction to Analytical Chemistry and Sensor Technology
Analytical chemistry is critical for understanding the chemical makeup of substances, allowing scientists to identify and quantify components in a sample (Zhang et al., 2011). Metal ion sensors are an important field of analytical chemistry, bringing both opportunities and limitations (Zhang et al., 2011). The sector has seen breakthroughs in wearable chemical sensors, which have considerably contributed to the improvement of analytical procedures. These sensors, whether electrochemical or optical, have altered on-body analytical chemistry, opening up new possibilities for real-time monitoring and analysis (Sempionatto et al., 2019).
Sensor technology has evolved to encompass bio and biomimetic receptors, expanding its applications in medical diagnostics, environmental monitoring, and food analysis (Козицинa et al., 2018). Furthermore, the use of conducting polymer-inorganic nanocomposites in gas sensors has shown promise, potentially increasing sensor technology capabilities (Yan et al., 2020). The development of optical cross-reactive sensor arrays has highlighted the significance of supramolecular analytical chemistry in sensor design and synthesis (Anzenbacher et al., 2010). Supramolecular chemistry has also played an important role in designing sensor arrays for distinguishing complicated systems, emphasizing the importance of molecular recognition in analytical techniques (Joyce et al., 2010).
Furthermore, green chemistry principles have been included into analytical procedures, reflecting the growing emphasis on sustainability in the field of chemistry (Koel & Kaljurand, 2006). DNA microarrays have opened up new potential for analytical chemists to contribute to technological progress, particularly in computational biology and DNA analysis (Wentzell & Karakach, 2005). Electrochemical biosensors using nanostructured carbon black have shown promise in a variety of domains, demonstrating the adaptability of sensor materials (Silva et al., 2017).
Furthermore, the use of cyclodextrins in analytical chemistry for molecular recognition has created new opportunities for non-chromatographic analytical applications (Szente & Szemán, 2013). The evaluation of sensor readings has become crucial, underlining the importance of accurate and trustworthy sensor data in analytical chemistry (Gauglitz, 2017). Selective protein sensing with aptamer-functionalized nanodevices has enabled accurate molecular detection, increasing our understanding of biological processes (Ren et al., 2020).
The integration of sensor technology with analytical chemistry has substantially advanced the field, allowing for precise and real-time analysis across a wide range of applications. From metal ion sensors to wearable chemical sensors and bio-inspired receptors, sensor technology is constantly improving analytical methodologies, opening up new avenues for study and practical applications.
2. Recent Advances in Sensor Technology for Analytical Chemistry
Recent improvements in sensor technology have had a substantial impact on analytical chemistry by increasing sensitivity, selectivity, and compactness. Wade and Bailey (2016) describe nanomaterial-based sensors as a significant advance that provide increased detection capabilities. Biosensors, a significant advancement in sensor technology, have enabled precise and fast analysis in a variety of sectors (Hassani & Dackermann, 2023). Wearable sensor technologies have gained popularity by offering real-time monitoring and analysis, altering the landscape of analytical chemistry (Regalado et al., 2019).
Over the last decade, there has been significant progress in the development of optical sensor technology for water quality monitoring, which allows for continuous measurement of pollution levels in both liquid and gas phases (Mizaikoff, 2003). Furthermore, the use of molecularly imprinted polymers to identify cancer biomarkers demonstrates advances in sensor technology for medical diagnostics (Pilvenyte et al., 2023). The use of graphene and its derivatives in electrochemical sensors to detect analytes in sweat highlights novel sensor design methodologies (Singh et al., 2022).
Furthermore, recent advances in the design of fluorescence sensing films have helped to improve analytical capacities (Peng, 2024). Rocío-Bautista et al. (2019) highlight the use of metal-organic frameworks in green analytical chemistry to produce revolutionary sensors. Furthermore, the development of triboelectric nanogenerator-based sensor systems emphasizes the incorporation of sensor technology into wearable devices for chemical and biological detection (Zhou et al., 2021).
The usage of covalent organic frameworks in electrochemical sensors represents a shift toward new materials for analytical chemistry applications (Xue et al., 2023). Furthermore, the use of all-in-fiber electrochemical sensing highlights the versatility and broad uses of electrochemical sensors (Richard et al., 2021). The creation of sustainable printed electrochemical platforms reflects an increasing emphasis on environmentally friendly analytical monitoring (Sfragano et al., 2020).
Recent breakthroughs in sensor technology have improved analytical chemistry by providing novel materials, designs, and procedures. From nanomaterial-based sensors to wearable devices and molecularly imprinted polymers, these advancements have pushed the field forward, allowing for more sensitive, selective, and long-lasting analytical solutions.
3. Challenges and Future Directions
The current difficulties in sensor technology for analytical chemistry include increasing long-term stability and decreasing interference. These problems are critical to improving the reliability and accuracy of sensor data. The integration of artificial intelligence (AI) for data analysis is a promising future development in sensor technology, providing increased capabilities for quickly processing and analyzing sensor data (Luo et al., 2019). AI can help find patterns, trends, and abnormalities in sensor data, resulting in more informative and actionable results for analytical chemistry applications.
Furthermore, the development of more sustainable sensor materials is an important future direction. Sustainable sensor materials can help to reduce environmental impact and promote eco-friendly practices in analytical chemistry. Researchers hope to develop sensors that are not only efficient and sensitive, but also environmentally friendly and cost-effective, by investigating novel materials and fabrication techniques (Tang et al., 2022).
The integration of AI and sensor technology creates new opportunities for improving analytical processes, real-time monitoring, and decision-making in a variety of domains. By tackling stability and interference issues while focusing on sustainable sensor materials, the future of sensor technology in analytical chemistry has enormous potential for increasing scientific research and practical applications.
References:
Anzenbacher, P., Lubal, P., Bucek, P., Palacios, M., & Kozelkova, M. (2010). A practical approach to optical cross-reactive sensor arrays. Chemical Society Reviews, 39(10), 3954. https://doi.org/10.1039/b926220m
Gauglitz, G. (2017). Analytical evaluation of sensor measurements. Analytical and Bioanalytical Chemistry, 410(1), 5-13. https://doi.org/10.1007/s00216-017-0624-z
Hassani, S. and Dackermann, U. (2023). A systematic review of advanced sensor technologies for non-destructive testing and structural health monitoring. Sensors, 23(4), 2204. https://doi.org/10.3390/s23042204
Joyce, L., Shabbir, S., & Anslyn, E. (2010). The uses of supramolecular chemistry in synthetic methodology development: examples of anion and neutral molecular recognition. Chemical Society Reviews, 39(10), 3621. https://doi.org/10.1039/b926224p
Koel, M. and Kaljurand, M. (2006). Application of the principles of green chemistry in analytical chemistry. Pure and Applied Chemistry, 78(11), 1993-2002. https://doi.org/10.1351/pac200678111993
Luo, Z., Hu, X., Tian, X., Luo, C., Xu, H., Li, Q., … & Chu, J. (2019). Structure-property relationships in graphene-based strain and pressure sensors for potential artificial intelligence applications. Sensors, 19(5), 1250. https://doi.org/10.3390/s19051250
Mizaikoff, B. (2003). Infrared optical sensors for water quality monitoring. Water Science & Technology, 47(2), 35-42. https://doi.org/10.2166/wst.2003.0079
Peng, H. (2024). Recent advances in construction strategies for fluorescence sensing films. The Journal of Physical Chemistry Letters, 15(4), 849-862. https://doi.org/10.1021/acs.jpclett.3c03130
Pilvenyte, G., Ratautaitė, V., Boguzaite, R., Ramanavičius, A., Viter, R., & Ramanavičius, S. (2023). Molecularly imprinted polymers for the determination of cancer biomarkers. International Journal of Molecular Sciences, 24(4), 4105. https://doi.org/10.3390/ijms24044105
Regalado, E., Ahmad, I., Bennett, R., D’Atri, V., Makarov, A., Humphrey, G., … & Guillarme, D. (2019). The emergence of universal chromatographic methods in the research and development of new drug substances. Accounts of Chemical Research, 52(7), 1990-2002. https://doi.org/10.1021/acs.accounts.9b00068
Ren, R., Wang, X., Cai, S., Zhang, Y., Korchev, Y., Ivanov, A., … & Edel, J. (2020). Selective sensing of proteins using aptamer functionalized nanopore extended field‐effect transistors. Small Methods, 4(11). https://doi.org/10.1002/smtd.202000356
Richard, I., Schyrr, B., Aiassa, S., Carrara, S., & Sorin, F. (2021). All-in-fiber electrochemical sensing. Acs Applied Materials & Interfaces, 13(36), 43356-43363. https://doi.org/10.1021/acsami.1c11593
Rocío‐Bautista, P., Taima‐Mancera, I., Pasán, J., & Pino, V. (2019). Metal-organic frameworks in green analytical chemistry. Separations, 6(3), 33. https://doi.org/10.3390/separations6030033
Sempionatto, J., Jeerapan, I., Krishnan, S., & Wang, J. (2019). Wearable chemical sensors: emerging systems for on-body analytical chemistry. Analytical Chemistry, 92(1), 378-396. https://doi.org/10.1021/acs.analchem.9b04668
Sfragano, P., Laschi, S., & Palchetti, I. (2020). Sustainable printed electrochemical platforms for greener analytics. Frontiers in Chemistry, 8. https://doi.org/10.3389/fchem.2020.00644
Silva, T., Moraes, F., Janegitz, B., & Fatibello‐Filho, O. (2017). Electrochemical biosensors based on nanostructured carbon black: a review. Journal of Nanomaterials, 2017, 1-14. https://doi.org/10.1155/2017/4571614
Singh, A., Ahmed, A., Sharma, A., & Arya, S. (2022). Graphene and its derivatives: synthesis and application in the electrochemical detection of analytes in sweat. Biosensors, 12(10), 910. https://doi.org/10.3390/bios12100910
Szente, L. and Szemán, J. (2013). Cyclodextrins in analytical chemistry: host–guest type molecular recognition. Analytical Chemistry, 85(17), 8024-8030. https://doi.org/10.1021/ac400639y
Tang, Y., Zhao, Y., & Liu, H. (2022). Room-temperature semiconductor gas sensors: challenges and opportunities. Acs Sensors, 7(12), 3582-3597. https://doi.org/10.1021/acssensors.2c01142
Wade, J. and Bailey, R. (2016). Applications of optical microcavity resonators in analytical chemistry. Annual Review of Analytical Chemistry, 9(1), 1-25. https://doi.org/10.1146/annurev-anchem-071015-041742
Wentzell, P. and Karakach, T. (2005). Dna microarrays: is there a role for analytical chemistry?. The Analyst, 130(10), 1331. https://doi.org/10.1039/b508677a
Xue, R., Liu, Y., Huang, S., & Yang, G. (2023). Recent progress of covalent organic frameworks applied in electrochemical sensors. Acs Sensors, 8(6), 2124-2148. https://doi.org/10.1021/acssensors.3c00269
Yan, Y., Yang, G., Xu, J., Zhang, M., Kuo, C., & Wang, S. (2020). Conducting polymer-inorganic nanocomposite-based gas sensors: a review. Science and Technology of Advanced Materials, 21(1), 768-786. https://doi.org/10.1080/14686996.2020.1820845
Zhang, X., Kong, R., & Lu, Y. (2011). Metal ion sensors based on dnazymes and related dna molecules. Annual Review of Analytical Chemistry, 4(1), 105-128. https://doi.org/10.1146/annurev.anchem.111808.073617
Zhou, Q., Pan, J., Deng, S., Xia, F., & Kim, T. (2021). Triboelectric nanogenerator‐based sensor systems for chemical or biological detection. Advanced Materials, 33(35). https://doi.org/10.1002/adma.202008276
Козицина, А., Свалова, Т., Малышева, Н., Охохонин, А., Vidrevich, M., & Брайнина, Х. (2018). Sensors based on bio and biomimetic receptors in medical diagnostic, environment, and food analysis. Biosensors, 8(2), 35. https://doi.org/10.3390/bios8020035