Advances in Polymer Chemistry: A Review
This review explores into the most recent advances in polymer chemistry, with an emphasis on novel synthesis processes, controlled polymerization methods, and the numerous applications of advanced polymers in biomedicine, electronics, and environmental sustainability.
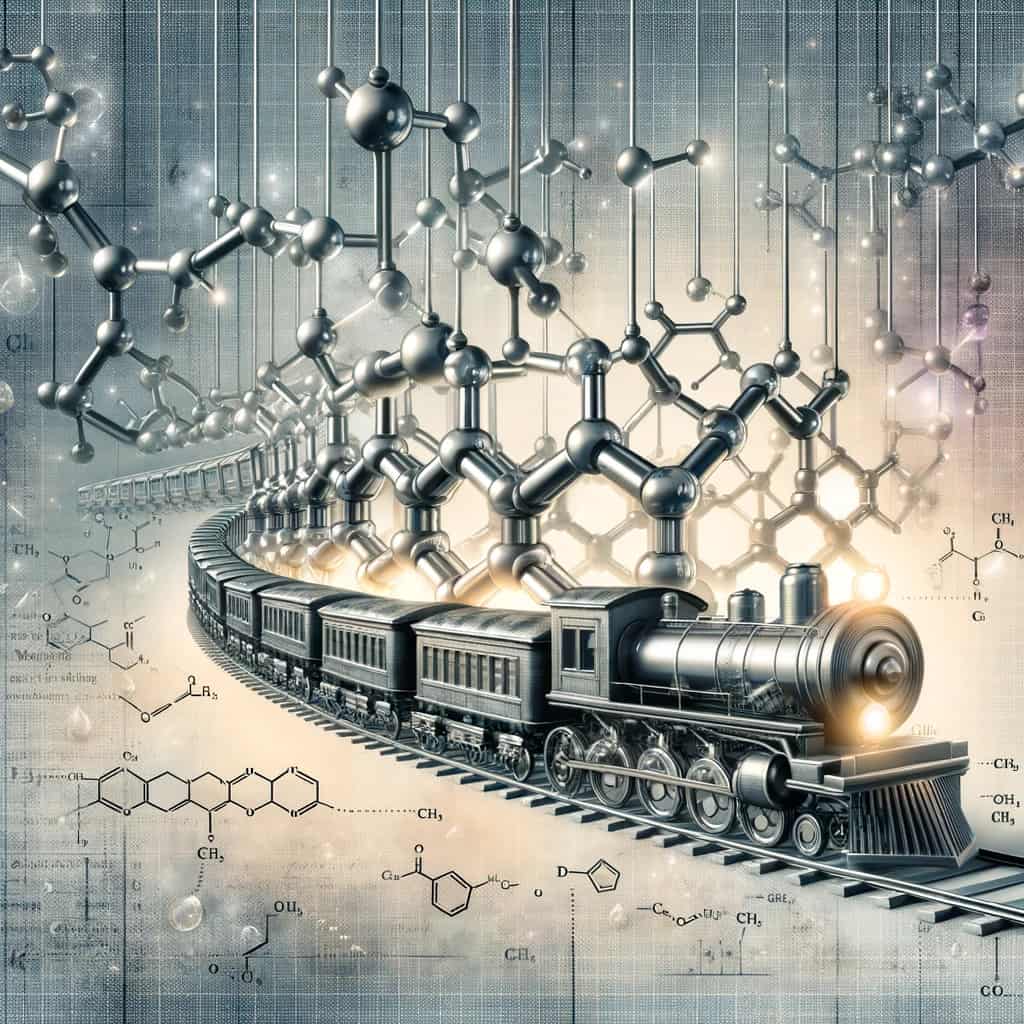
1. Introduction to Polymer Chemistry
Polymer chemistry is an important discipline in scientific research and technological applications, with issues including polymerization mechanisms, molecular weight distribution, and structural variety. Researchers have investigated numerous elements of polymer chemistry in order to stimulate innovation and applications.
One important field of research is the creation of supramolecular polymers using host-guest molecular recognition motifs (Dong et al., 2014). These polymers display features such as stimulus responsiveness and self-healing, which improve polymer functioning. Furthermore, the use of polyurethane chemistry has resulted in the production of a diversified polymeric material with a wide range of properties suitable for a variety of applications (Alinejad et al., 2019).
Multicomponent polymerizations have emerged as an effective method for producing multifunctional polymers with distinct properties (Su et al., 2020; Wang et al., 2022; Su et al., 2021). These approaches provide up new possibilities for creating and manipulating functional polymer materials, resulting in unique applications. Combinatorial techniques to post-polymerization modification have also been investigated to improve medicinal delivery systems (Zhong et al., 2018).
The use of mass spectrometry and hyphenated techniques has facilitated polymer architecture analysis, assisting with data interpretation and expediting polymer science research (Crotty et al., 2016). Furthermore, the emergence of sequence-defined nucleic acid polymers has prompted efforts to apply similar ideas to synthetic polymers, allowing for the creation of highly functionalized polymers (Chen et al., 2018).
Researchers have investigated the effect of polymers on crystal growth rates, focusing on their role in regulating the crystallization of diverse substances (Ilevbare et al., 2013). Furthermore, developing polymers from renewable resources is both a challenge and an opportunity for producing cost-effective and competitive materials (Gregory et al., 2017).
Polymer chemistry is a dynamic field that is always evolving as new discoveries and applications emerge. Researchers are broadening the possibilities with polymers by synthesising supramolecular polymers and investigating various polymerization processes. By using breakthrough approaches and materials, the future of polymer chemistry holds considerable promise for addressing scientific challenges and fostering technological innovation.
2. Recent Innovations in Polymer Synthesis
Recent breakthroughs in polymer synthesis have been greatly influenced by advances in controlled/living polymerization technologies, which provide exact control over polymer architecture, functionality, and composition. These strategies have changed the profession by making it easier to create well-defined polymers with specific features.
Boyer et al. (2011) reported a significant advancement in the synthesis of complex multiblock copolymers via repeated Cu(0)-mediated radical polymerization. This method demonstrated the efficacy of controlled/living radical polymerization in making well-defined polymeric structures, such as copolymers and block copolymers. Furthermore, the combination of live cationic polymerization and other polymerization processes has resulted in significant advances in block copolymer production (Dey et al., 2021).
Polymeropoulos et al. (2017) report that controlled/living polymerization systems have enabled the creation of polymers with complex topologies, including normal and μ-star polymers. These systems have permitted the polymerization of monomers that were previously difficult to polymerize using traditional methods, expanding the scope of polymer synthesis.
Living/controlled polymerization technologies, such as atom-transfer radical polymerization (ATRP) and reversible addition-fragmentation transfer polymerization (RAFT), have been critical in developing amphiphilic polymer-oligonucleotide nanomaterials (Sun et al., 2019). These approaches have provided precise control over polymer-oligonucleotide conjugates, opening up new possibilities in nanotechnology and materials research.
Furthermore, recent advances in RAFT polymerization have revealed novel starting mechanisms with potential applications in the optoelectronic domain (Tian et al., 2018). These advances highlight the ongoing growth of polymer synthesis techniques and their numerous applications in cutting-edge industries.
The synthesis of core-cross-linked multiarm star polyethylenes using palladium-catalyzed ethylene “living” polymerization and ATRP demonstrates the adaptability and range of controlled radical polymerization techniques (Liu et al., 2011). These approaches have substantially expanded the possibilities for creating well-defined star polymers from multiple monomer sources.
Recent advances in polymer synthesis have been primarily driven by the development of controlled/living polymerization technologies. These techniques have enabled precise control over polymer design, functioning, and composition, resulting in the creation of unique materials with specific features. The ongoing advancement of polymer synthesis techniques holds the promise of future advances in materials science and a wide range of application sectors.
3. Applications of Advanced Polymers
In recent years, innovative polymers have made important contributions to a variety of sectors, including biology. Biocompatible polymers have played a critical role in drug delivery systems, with alginate electrospinning demonstrating uses in biomedicine and packaging Wróblewska-Krepsztul et al. (2019). The combination of CeO2 nanoparticles and polymers has resulted in the creation of composite biomaterials for medicinal applications such as tissue regeneration and medication delivery (Shcherbakov et al., 2021). Polymers have also been widely used in medication administration, cancer treatment, and antimicrobial applications, demonstrating their versatility in healthcare (Yu et al., 2016). Furthermore, the integration of electrically conductive nanoparticles in hydrogels has allowed for applications in biomedicine, tissue engineering, and soft electronics (KOUGKOLOS et al., 2023). Cyclodextrin polymers have demonstrated promise in a variety of domains, including absorption, catalysis, and biomedicine, highlighting their versatility and potential for biological applications (Qie et al., 2020).
These advances illustrate the wide range of applications for improved polymers in biomedicine, from drug delivery to tissue engineering, as well as their critical role in the progress of healthcare technologies.
References:
Alinejad, M., Henry, C., Nikafshar, S., Gondaliya, A., Bagheri, S., Chen, N., … & Nejad, M. (2019). Lignin-based polyurethanes: opportunities for bio-based foams, elastomers, coatings and adhesives. Polymers, 11(7), 1202. https://doi.org/10.3390/polym11071202
Boyer, C., Soeriyadi, A., Zetterlund, P., & Whittaker, M. (2011). Synthesis of complex multiblock copolymers via a simple iterative cu(0)-mediated radical polymerization approach. Macromolecules, 44(20), 8028-8033. https://doi.org/10.1021/ma201529j
Chen, Z., Lichtor, P., Berliner, A., Chen, J., & Liu, D. (2018). Evolution of sequence-defined highly functionalized nucleic acid polymers. Nature Chemistry, 10(4), 420-427. https://doi.org/10.1038/s41557-018-0008-9
Crotty, S., Gerişlioğlu, S., Endres, K., Wesdemiotis, C., & Schubert, U. (2016). Polymer architectures via mass spectrometry and hyphenated techniques: a review. Analytica Chimica Acta, 932, 1-21. https://doi.org/10.1016/j.aca.2016.05.024
Dey, A., Haldar, U., & De, P. (2021). Block copolymer synthesis by the combination of living cationic polymerization and other polymerization methods. Frontiers in Chemistry, 9. https://doi.org/10.3389/fchem.2021.644547
Dong, S., Zheng, B., Wang, F., & Huang, F. (2014). Supramolecular polymers constructed from macrocycle-based host–guest molecular recognition motifs. Accounts of Chemical Research, 47(7), 1982-1994. https://doi.org/10.1021/ar5000456
Gregory, G., Lopez‐Vidal, E., & Buchard, A. (2017). Polymers from sugars: cyclic monomer synthesis, ring-opening polymerisation, material properties and applications. Chemical Communications, 53(14), 2198-2217. https://doi.org/10.1039/c6cc09578j
Ilevbare, G., Liu, H., Edgar, K., & Taylor, L. (2013). Impact of polymers on crystal growth rate of structurally diverse compounds from aqueous solution. Molecular Pharmaceutics, 10(6), 2381-2393. https://doi.org/10.1021/mp400029v
KOUGKOLOS, G., Golzio, M., Laudebat, L., Valdez-Nava, Z., & Flahaut, E. (2023). Hydrogels with electrically conductive nanomaterials for biomedical applications. Journal of Materials Chemistry B, 11(10), 2036-2062. https://doi.org/10.1039/d2tb02019j
Liu, P., Landry, E., Ye, Z., Joly, H., Wang, W., & Li, B. (2011). “arm-first” synthesis of core-cross-linked multiarm star polyethylenes by coupling palladium-catalyzed ethylene “living” polymerization with atom-transfer radical polymerization. Macromolecules, 44(11), 4125-4139. https://doi.org/10.1021/ma2003954
Polymeropoulos, G., Ζάψας, Γ., Ντέτσικας, Κ., Bilalis, P., Gnanou, Y., & Hadjichristidis, N. (2017). 50th anniversary perspective: polymers with complex architectures. Macromolecules, 50(4), 1253-1290. https://doi.org/10.1021/acs.macromol.6b02569
Qie, S., Hao, Y., Liu, Z., Wang, J., & Xi, J. (2020). Advances in cyclodextrin polymers and their applications in biomedicine. Acta Chimica Sinica, 78(3), 232. https://doi.org/10.6023/a20010006
Shcherbakov, A., Reukov, V., Yakimansky, А., Краснопеева, Е., Иванова, О., Попов, А., … & Иванов, В. (2021). Ceo2 nanoparticle-containing polymers for biomedical applications: a review. Polymers, 13(6), 924. https://doi.org/10.3390/polym13060924
Su, X., Gao, Q., Wang, D., Han, T., & Tang, B. (2020). One‐step multicomponent polymerizations for the synthesis of multifunctional aie polymers. Macromolecular Rapid Communications, 42(6). https://doi.org/10.1002/marc.202000471
Su, X., Han, T., Li, H., Wang, D., & Tang, B. (2021). Facile multicomponent polymerizations toward multifunctional heterochain polymers with α,β-unsaturated amidines. Macromolecules, 54(21), 9906-9918. https://doi.org/10.1021/acs.macromol.1c01623
Sun, H., Lu, Y., Thompson, M., Schara, S., Cao, W., Choi, W., … & Gianneschi, N. (2019). Recent advances in amphiphilic polymer–oligonucleotide nanomaterials via living/controlled polymerization technologies. Bioconjugate Chemistry, 30(7), 1889-1904. https://doi.org/10.1021/acs.bioconjchem.9b00166
Tian, X., Ding, J., Zhang, B., Qiu, F., Zhuang, X., & Chumakov, Y. (2018). Recent advances in raft polymerization: novel initiation mechanisms and optoelectronic applications. Polymers, 10(3), 318. https://doi.org/10.3390/polym10030318
Wang, X., Han, T., Gong, J., Alam, P., Zhang, H., Lam, J., … & Tang, B. (2022). Diversity-oriented synthesis of functional polymers with multisubstituted small heterocycles by facile stereoselective multicomponent polymerizations. Macromolecules, 55(11), 4389-4401. https://doi.org/10.1021/acs.macromol.2c00319
Wróblewska-Krepsztul, J., Rydzkowski, T., Michalska-Pożoga, I., & Thakur, V. (2019). Biopolymers for biomedical and pharmaceutical applications: recent advances and overview of alginate electrospinning. Nanomaterials, 9(3), 404. https://doi.org/10.3390/nano9030404
Yu, Q., Ista, L., Gu, R., Zauscher, S., & López, G. (2016). Nanopatterned polymer brushes: conformation, fabrication and applications. Nanoscale, 8(2), 680-700. https://doi.org/10.1039/c5nr07107k
Zhong, Y., Zeberl, B., Wang, X., & Luo, J. (2018). Combinatorial approaches in post-polymerization modification for rational development of therapeutic delivery systems. Acta Biomaterialia, 73, 21-37. https://doi.org/10.1016/j.actbio.2018.04.010