Advances in Bioconjugate Biomaterials for Biomedical Applications
This review looks at the synthesis and application of bioconjugate biomaterials, focusing on their function in drug delivery, tissue engineering, and regenerative medicine, as well as their promise to improve individualized medical therapies.
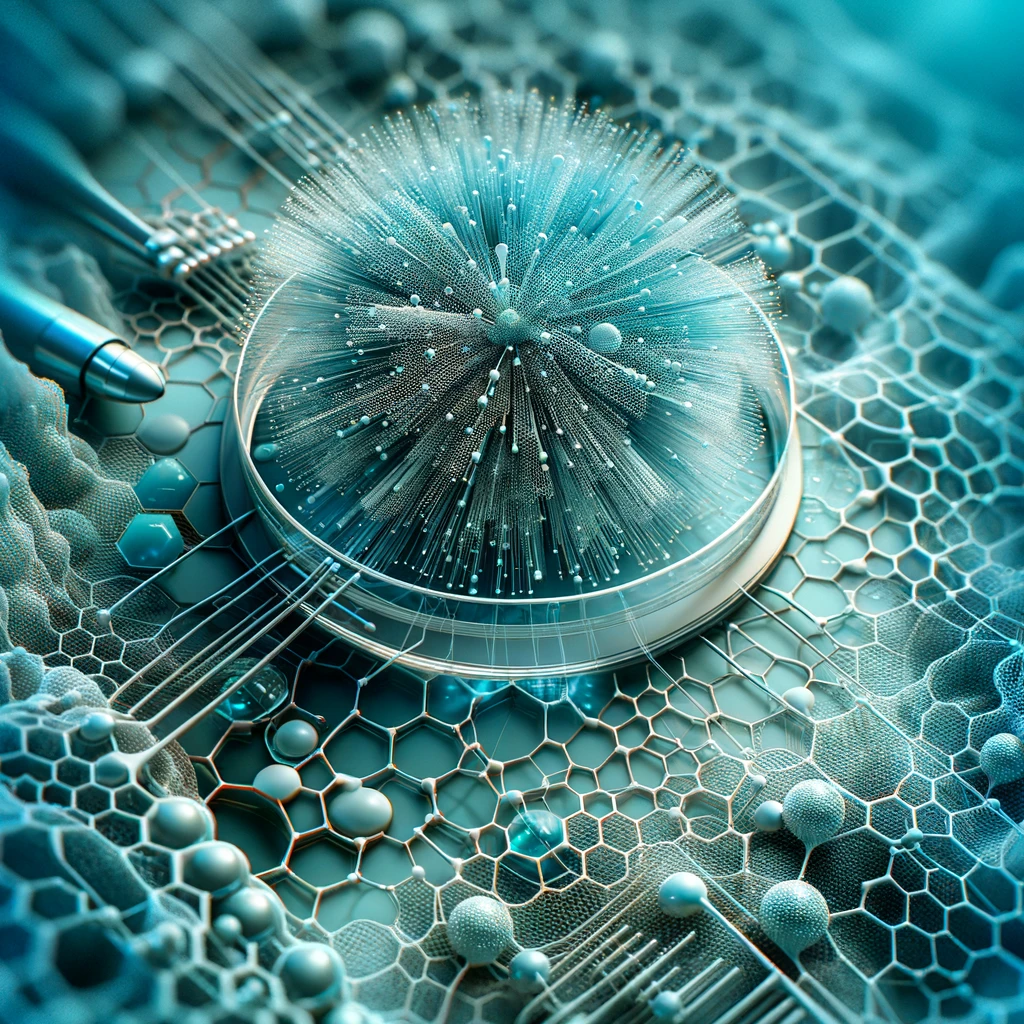
Introduction to Bioconjugate Biomaterials
Bioconjugate biomaterials are important in a variety of biomedical applications due to their unique characteristics and adaptability. These biomaterials entail changing biomacromolecules by attaching polymers or other molecules, resulting in the creation of novel polymeric biomaterials (Cobo et al., 2014). One typical way is to use addition chemistries like maleimide-thiol adducts, which are widely used in bioconjugation processes (Baldwin & Kiick, 2011). Furthermore, the investigation of alternative materials such as linear polyglycerol as a replacement for polyethylene glycol (PEG) has opened up new avenues for bioconjugation and the creation of biodegradable biomaterials (Thomas et al., 2014).
Bioconjugated hydrogels have emerged as potential materials for tissue engineering and regenerative medicine, providing a framework for their production, modification, and clinical use (Ahadian et al., 2015). Collagen-like peptide bioconjugates have demonstrated tremendous potential in a variety of biological applications, underlining the advancements and opportunity for incorporating these peptides into biomaterial design (Luo and Kiick, 2017). Furthermore, the use of click chemistry in the synthesis and deployment of regenerative biomaterials has resulted in simple aqueous-based methods for hydrogel synthesis, surface immobilization, and 3D patterning, increasing their applicability in tissue engineering (Nimmo & Shoichet, 2011).
Bioconjugation research now includes the utilization of carbon nanomaterials such as fullerenes, quantum dots, nanotubes, nanofibers, and graphene in biomedical applications (Gaur et al., 2021). Nanoparticle-aptamer bioconjugates have been studied for cancer treatment, demonstrating its potential in targeted drug delivery systems (Farokhzad et al., 2006). Furthermore, the development of novel biomaterials such as luminous carbon dots for drug delivery applications exemplifies the innovative approaches being pursued in biomaterial design (Ghataty et al., 2023).
Bioconjugate biomaterials are a multifaceted and dynamic topic with important implications for biomedical research and applications. Researchers are paving the way for the development of next-generation biomaterials with better capabilities and specialized properties for a wide range of biomedical applications by utilizing sophisticated synthesis processes, new materials, and bioconjugation strategies.
Synthesis and Functionalization Techniques
Bioconjugate biomaterials are synthesized using a variety of processes, each of which plays an important role in modifying the materials’ characteristics and functionality. One typical strategy is to copolymerize vinyl-functionalized biomacromolecules with responsive co-monomers, resulting in smart hybrid materials with programmable properties (Cobo et al., 2014). Furthermore, polymer-protein hybrids have been created using several coupling processes and polymerization methods, providing a diverse platform for bioconjugate synthesis (Ju et al., 2018).
Surface functionalization is an important step in improving biomaterials’ biocompatibility and functioning. Bioconjugation has been achieved by modifying nanoparticles with reactive groups such as carboxyl and amine groups, allowing for the covalent attachment of biomolecules by standardized bioconjugation procedures (Oliveira et al., 2019). Furthermore, the creation of zwitterionic diblock copolymers with cleavable biotin groups has enabled the production of bioconjugates via biotin-streptavidin coupling, demonstrating an approach for customized bioconjugation (Wang et al., 2017).
Bioconjugation procedures, which include the covalent binding of different molecules, have been widely used to build stable and functional biomolecule combinations for a variety of biomedical applications (Ahadian et al., 2015). Living radical polymerization methods have been used to synthesize polymer-protein/peptide bioconjugates, exhibiting their versatility and precision (Nicolas et al., 2007). Furthermore, the self-assembly of well-defined polymer-protein conjugates has sparked great interest in applications such as drug delivery and protein stability, emphasizing the significance of regulated bioconjugation approaches (Hou et al., 2018).
The synthesis of bioconjugate biomaterials via various processes, as well as the strategic surface functionalization of these materials, are critical in adapting their properties to specific biological applications. Researchers can use advanced bioconjugation techniques and surface modification tactics to create bioconjugate biomaterials with improved functions, biocompatibility, and targeted applications in a variety of biomedicine domains.
Applications in Medicine and Research
Bioconjugate biomaterials are used in a variety of medical and research applications, including medication delivery systems, diagnostic imaging, and tissue engineering. Quantum dot bioconjugates have shown promise in cellular labeling, deep-tissue imaging, and fluorescence resonance energy transfer, providing a wide range of applications in imaging, labeling, and sensing Medintz et al. (2005). In tissue engineering and regenerative medicine, microscale biomaterials inspired by early embryo development have been used to reproduce complex biological systems, increasing their potential for a variety of biomedical applications (He, 2016). Gelatin-based treatments have advanced regulated drug administration, prosthetics, and tissue engineering, demonstrating the growing role of biomaterials in medicine (Echave et al., 2019).
Furthermore, biomaterial characteristics have a significant impact on bone regeneration, highlighting the importance of biomaterials in tissue engineering as biological alternatives for tissue repair and regeneration (Zhu et al., 2021). Photocleavable biomaterials and bioconjugates have enabled applications in tissue engineering, cell culture, and targeted medicines delivery (Sun et al., 2022). Furthermore, synthetic protein-iron oxide hybrid biomaterials have been developed for MRI-traceable drug encapsulation, allowing implant functionalization for drug delivery and imaging applications (Hill et al., 2022).
Future directions in this research include the development of smart biomaterials with variable properties for tissue engineering, drug delivery systems, medical devices, and immunological engineering (Kowalski et al., 2018). The integration of cancer imaging and therapy on nanoshell-based platforms is a novel approach to cancer treatment (Loo et al. 2005). Furthermore, the interaction between biomaterials and immune responses is a critical field of research, with biomaterial design expected to play an increasingly important role in a variety of medicinal applications (Okawa et al., 2020).
To summarize, bioconjugate biomaterials have revolutionized the landscape of medicine and research, offering novel options for medication delivery, tissue engineering, and diagnostic imaging. With continued advances in biomaterial design, synthesis methodologies, and functionalization tactics, the future holds enormous promise for breakthroughs in personalized medicine, regenerative therapies, and targeted treatment modalities.
Reference
Ahadian, S., Sadeghian, R., Salehi, S., Ostrovidov, S., Bae, H., & Ramalingam, M. (2015). Bioconjugated hydrogels for tissue engineering and regenerative medicine. Bioconjugate Chemistry, 26(10), 1984-2001. https://doi.org/10.1021/acs.bioconjchem.5b00360
Baldwin, A. and Kiick, K. (2011). Tunable degradation of maleimide–thiol adducts in reducing environments. Bioconjugate Chemistry, 22(10), 1946-1953. https://doi.org/10.1021/bc200148v
Cobo, I., Li, M., Sumerlin, B., & Perrier, S. (2014). Smart hybrid materials by conjugation of responsive polymers to biomacromolecules. Nature Materials, 14(2), 143-159. https://doi.org/10.1038/nmat4106
Farokhzad, O., Karp, J., & Langer, R. (2006). Nanoparticle–aptamer bioconjugates for cancer targeting. Expert Opinion on Drug Delivery, 3(3), 311-324. https://doi.org/10.1517/17425247.3.3.311
Gaur, M., Misra, C., Yadav, A., Swaroop, S., Maolmhuaidh, F., Bechelany, M., … & Barhoum, A. (2021). Biomedical applications of carbon nanomaterials: fullerenes, quantum dots, nanotubes, nanofibers, and graphene. Materials, 14(20), 5978. https://doi.org/10.3390/ma14205978
Ghataty, D., Amer, R., Amer, M., Rahman, M., & Shamma, R. (2023). Green synthesis of highly fluorescent carbon dots from bovine serum albumin for linezolid drug delivery as potential wound healing biomaterial: bio-synergistic approach, antibacterial activity, and in vitro and ex vivo evaluation. Pharmaceutics, 15(1), 234. https://doi.org/10.3390/pharmaceutics15010234
Luo, T. and Kiick, K. (2017). Collagen-like peptide bioconjugates. Bioconjugate Chemistry, 28(3), 816-827. https://doi.org/10.1021/acs.bioconjchem.6b00673
Nimmo, C. and Shoichet, M. (2011). Regenerative biomaterials that “click”: simple, aqueous-based protocols for hydrogel synthesis, surface immobilization, and 3d patterning. Bioconjugate Chemistry, 22(11), 2199-2209. https://doi.org/10.1021/bc200281k
Thomas, A., Müller, S., & Frey, H. (2014). Beyond poly(ethylene glycol): linear polyglycerol as a multifunctional polyether for biomedical and pharmaceutical applications. Biomacromolecules, 15(6), 1935-1954. https://doi.org/10.1021/bm5002608
Ahadian, S., Sadeghian, R., Salehi, S., Ostrovidov, S., Bae, H., & Ramalingam, M. (2015). Bioconjugated hydrogels for tissue engineering and regenerative medicine. Bioconjugate Chemistry, 26(10), 1984-2001. https://doi.org/10.1021/acs.bioconjchem.5b00360
Cobo, I., Li, M., Sumerlin, B., & Perrier, S. (2014). Smart hybrid materials by conjugation of responsive polymers to biomacromolecules. Nature Materials, 14(2), 143-159. https://doi.org/10.1038/nmat4106
Hou, W., Lin, W., Liu, L., & Zhao, H. (2018). Surface coassembly of polymer brushes and polymer–protein bioconjugates: an efficient approach to the purification of bioconjugates under mild conditions. Biomacromolecules, 19(11), 4463-4471. https://doi.org/10.1021/acs.biomac.8b01355
Ju, Y., Zhang, Y., & Zhao, H. (2018). Fabrication of polymer–protein hybrids. Macromolecular Rapid Communications, 39(7). https://doi.org/10.1002/marc.201700737
Nicolas, J., Mantovani, G., & Haddleton, D. (2007). Living radical polymerization as a tool for the synthesis of polymer‐protein/peptide bioconjugates. Macromolecular Rapid Communications, 28(10), 1083-1111. https://doi.org/10.1002/marc.200700112
Oliveira, J., Prado, A., Keijok, W., Antunes, P., Yapuchura, E., & Guimarães, M. (2019). Impact of conjugation strategies for targeting of antibodies in gold nanoparticles for ultrasensitive detection of 17β-estradiol. Scientific Reports, 9(1). https://doi.org/10.1038/s41598-019-50424-5
Wang, J., Wang, L., Ji, X., Liu, L., & Zhao, H. (2017). Synthesis of zwitterionic diblock copolymers with cleavable biotin groups at the junction points and fabrication of bioconjugates by biotin–streptavidin coupling. Macromolecules, 50(6), 2284-2295. https://doi.org/10.1021/acs.macromol.6b02665
Echave, M., Hernáez‐Moya, R., Iturriaga, L., Pedraz, J., Lakshminarayanan, R., Dolatshahi-Pirouz, A., … & Orive, G. (2019). Recent advances in gelatin-based therapeutics. Expert Opinion on Biological Therapy, 19(8), 773-779. https://doi.org/10.1080/14712598.2019.1610383
He, X. (2016). Microscale biomaterials with bioinspired complexity of early embryo development and in the ovary for tissue engineering and regenerative medicine. Acs Biomaterials Science & Engineering, 3(11), 2692-2701. https://doi.org/10.1021/acsbiomaterials.6b00540
Hill, L., Britton, D., Jihad, T., Punia, K., Xie, X., Delgado-Fukushima, E., … & Montclare, J. (2022). Engineered protein–iron oxide hybrid biomaterial for mri-traceable drug encapsulation. Molecular Systems Design & Engineering, 7(8), 915-932. https://doi.org/10.1039/d2me00002d
Kowalski, P., Bhattacharya, C., Afewerki, S., & Langer, R. (2018). Smart biomaterials: recent advances and future directions. Acs Biomaterials Science & Engineering, 4(11), 3809-3817. https://doi.org/10.1021/acsbiomaterials.8b00889
Loo, C., Lowery, A., Halas, N., West, J., & Drezek, R. (2005). Immunotargeted nanoshells for integrated cancer imaging and therapy. Nano Letters, 5(4), 709-711. https://doi.org/10.1021/nl050127s
Medintz, I., Uyeda, H., Goldman, E., & Mattoussi, H. (2005). Quantum dot bioconjugates for imaging, labelling and sensing. Nature Materials, 4(6), 435-446. https://doi.org/10.1038/nmat1390
Okawa, M., Sakoda, M., Ohta, S., Hasegawa, K., Yatomi, Y., & Ito, T. (2020). The balance between the hemostatic effect and immune response of hyaluronan conjugated with different chain lengths of inorganic polyphosphate. Biomacromolecules, 21(7), 2695-2704. https://doi.org/10.1021/acs.biomac.0c00390
Sun, F., Yu, W., Wang, Q., Wang, X., Pu, Y., Feng, W., … & Zhang, D. (2022). Self-illuminating triggered release of therapeutics from photocleavable nanoprodrug for the targeted treatment of breast cancer. Acs Applied Materials & Interfaces, 14(7), 8766-8781. https://doi.org/10.1021/acsami.1c21665
Zhu, Y., Goh, C., & Shrestha, A. (2021). Biomaterial properties modulating bone regeneration. Macromolecular Bioscience, 21(4). https://doi.org/10.1002/mabi.202000365