Innovations in Asymmetric Catalysis
Recent developments in asymmetric catalysis are examined in this review, with an emphasis on novel catalysts, processes, and applications. It draws attention to creative methods that improve enantioselectivity and productivity when creating chiral compounds.
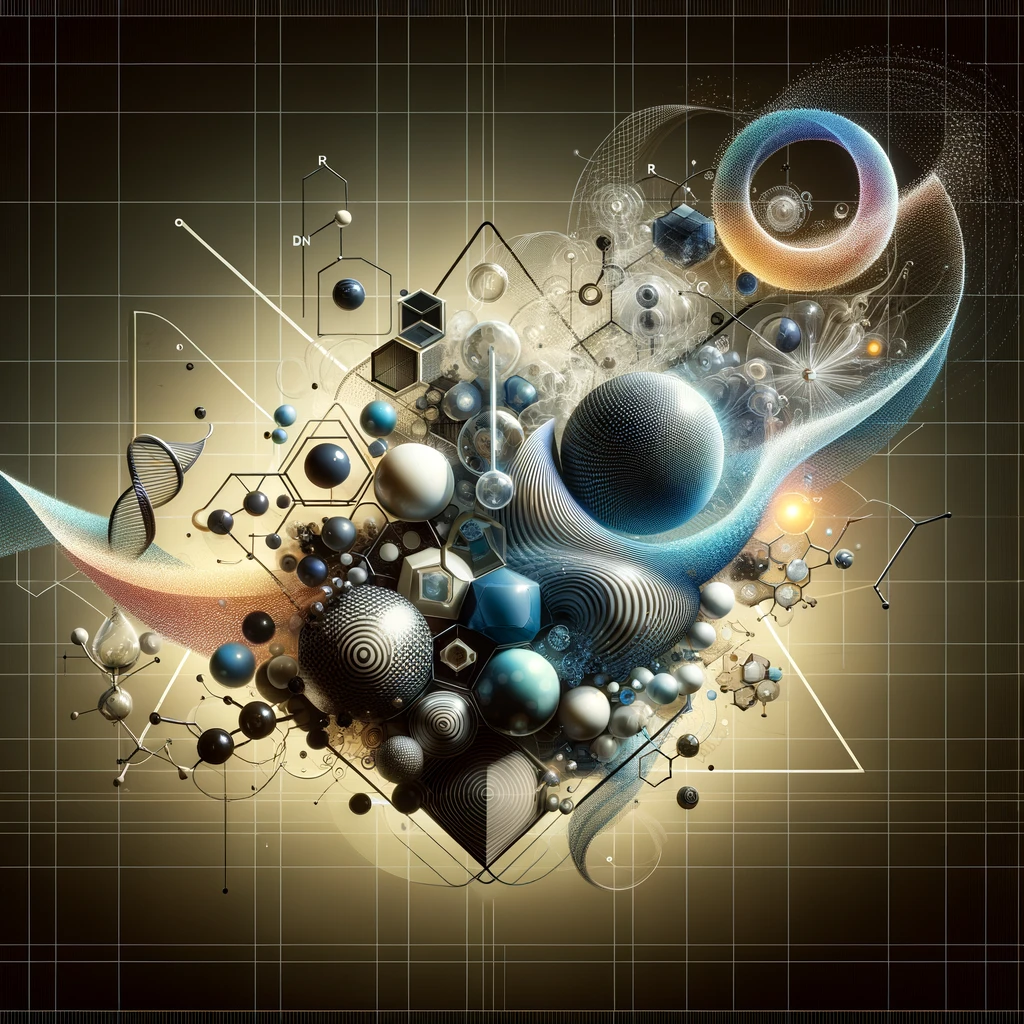
Introduction to Asymmetric Catalysis
Due to the growing need for chiral compounds, the fundamental discipline of chemistry known as asymmetric catalysis is concerned with producing optically pure materials. High selectivity molecules can be created with this technique, yielding substances that are enantiomerically pure. Asymmetric catalysis is important for many industries, but it’s especially important for the pharmaceutical, agrochemical, and fine chemical industries.
The potential of asymmetric catalysis to yield chiral medications with improved efficacy and fewer adverse effects makes it significant in the pharmaceutical industry. Pharmaceuticals that are enantiomerically pure are essential because they guarantee that the medication interacts specifically with its target, improving therapeutic results. Asymmetric catalysis, which permits the selective synthesis of chiral centers and affects the biological activity of the compounds, allows for this level of accuracy in drug design (Huo et al., 2014).
Asymmetric catalysis plays a crucial role in the creation of chiral insecticides and herbicides in the agrochemical sector. Agrochemicals’ chirality is essential to their efficacy since their enantiomeric versions can have distinct biological effects. Asymmetric catalysis makes it possible to produce single enantiomers, which guarantees that the agrochemicals minimize their negative effects on the environment while targeting certain pests or weeds (Huo et al., 2014).
Asymmetric catalysis is crucial in the field of fine chemicals because it allows for the synthesis of complex chiral compounds that are employed in tastes, perfumes, and specialty chemicals, among other applications. Asymmetric catalysis’s capacity to regulate these compounds’ stereochemistry is essential to guaranteeing the intended features and functions of the finished goods. In order to satisfy the needs of many industries, asymmetric catalysis provides an economical and effective method of obtaining fine compounds that are enantiomerically pure (Huo et al., 2014).
Asymmetric catalysis is essential to the synthesis of highly selective chiral compounds, which are used in the fine chemical, agrochemical, and pharmaceutical industries. Researchers can improve medicine development, agriculture, and the synthesis of specialty chemicals by controlling the characteristics and activities of molecules through the use of asymmetric catalysis.
Key Catalysis
Selective chiral chemical manufacturing is made possible via asymmetric synthesis, which uses a variety of catalysts and processes. The field has made substantial progress recently due to advances that provide fresh understanding of the mechanisms involved in generating high enantioselectivity and catalyst design.
Enantioselective hydrogenation is a prominent process in asymmetric synthesis that has drawn interest because of its potential for effectively generating chiral molecules. Asymmetric hydrogenation may be made more economically and environmentally friendly with the help of recent advancements like the utilization of transition metal catalysts that are abundant on Earth, such as nickel (Li et al., 2021).
As another crucial method, enantiomerically enhanced epoxides are formed through asymmetric epoxidation. Asymmetric synthesis can be made more versatile by using catalysts such as iminium salts produced from binaphthalene, which have been created to support highly enantioselective asymmetric epoxidation processes (Page et al., 2009).
Furthermore, a potent tactic in asymmetric synthesis is the idea of bifunctional catalysis. This idea allows for the simultaneous activation of several process components by catalysts, improving selectivity and efficiency. Complex catalytic systems have the potential to propel advances in chiral molecule synthesis, as evidenced by the exploration of multimetallic systems as efficient catalysts in asymmetric bifunctional catalysis (Shibasaki et al., 2009).
Organocatalysts, with their many catalyst designs, reduced toxicity, and ease of use, have also made important contributions to asymmetric synthesis. The toolkit of catalysts available for chiral compound synthesis has been expanded by the development of effective catalysts for a variety of asymmetric reactions, which have been made possible by designing organocatalysts with specific structural features, such as phosphinic acid catalysts with close stereogenicity (Fujii et al., 2019).
In addition, obtaining strong enantioselectivity in asymmetric synthesis has been made possible by the use of chiral metal complexes as catalysts. One successful application of optically active ligands in driving crucial processes with excellent stereoselectivity is the asymmetric synthesis of muscone using the Cp*Ru(PN) complex-catalyzed isomerization of allylic alcohols (Ito et al., 2005).
Recent developments in asymmetric synthesis have brought to light the variety of catalysts and processes used to attain excellent selectivity in the synthesis of chiral compounds. Through the use of organocatalysts and chiral metal complexes, enantioselective hydrogenation, asymmetric epoxidation, and other advances, asymmetric catalysis is constantly evolving to produce optically pure molecules in more sustainable and effective ways.
Applications and Future Directions
Asymmetric catalysis is a vital step in the synthesis of more complex molecules, including active pharmaceutical ingredients (APIs), and it offers a reliable way to create compounds that are enantiomerically pure. Asymmetric catalysis is widely used in the pharmaceutical industry to create chiral medications with higher efficacy and fewer negative effects. Asymmetric catalysis provides precise control over the stereochemistry of molecules, which is essential for pharmaceutical compounds to have biological activity and selectivity, which in turn leads to improved therapeutic outcomes (Huo et al., 2014).
Additionally, biologically active substances including amines, amides, and amino nitroalkanes are produced via asymmetric catalysis. Asymmetric catalysis is useful for the synthesis of complex molecules because of its versatility and importance. Recent developments in enantioselective reactions and asymmetric hydrogenation have made it easier to synthesize these valuable intermediates with high yields and remarkable enantioselectivity (Palo‐Nieto et al., 2016; Zhou et al., 2013).
Scholars are examining novel approaches like recyclable catalyst design and integrated heterogeneous metal/enzymatic catalysis as means of achieving ecologically friendly and effective catalytic processes. These strategies seek to increase catalytic efficiency and reduce waste generation in order to improve the sustainability of chemical processes. For instance, using recyclable organocatalysts and magnetic composites, which are heterogeneous catalysts, could lead to the development of more environmentally friendly synthesis routes with better catalyst recovery and reusability (Cho, 2009; Dohi, 2010; Wang et al., 2023).
Asymmetric synthesis could also move toward more environmentally friendly methods thanks to the development of cutting-edge catalytic methods including enantioselective and chemo-hydrogenation, asymmetric amination, and organoradical-based oxidations. Researchers advocate for the use of environmentally benign catalytic systems while attempting to address current synthesis challenges through the focus on site-selective transformations, efficient enantioselective reactions, and controlled alkane C-H oxidations (Sawada et al., 2000; Zhao, 2023; Chen et al., 2022).
Future studies in asymmetric catalysis might investigate innovative catalytic systems such mechanochemical methods, ionic liquid-based catalysis, and photoinduced processes. These strategies provide exceptional chances to create sustainable and ecologically friendly methods for organic synthesis, opening the door to more effective and ecologically responsible ways to synthesize complex compounds (Ji et al., 2021; Li et al., 2021; Li et al., 2021).
To sum up, asymmetric catalysis is essential to the synthesis of complex molecules and APIs because it allows for fine control over stereochemistry and the creation of enantiomerically pure substances. Researchers can further advance the field of asymmetric synthesis towards more environmentally friendly and productive procedures, hence aiding in the development of novel medications and fine chemicals, by adopting sustainable practices and innovative catalytic methodologies.
References:
Chen, Z., Annamalai, P., Bai, R., Hu, Y., Badsara, S., Huang, K., … & Lee, C. (2022). Cesium carbonate-catalyzed synthesis of phosphorothioates via s-phosphination of thioketones. Chemical Communications, 58(78), 11001-11004. https://doi.org/10.1039/d2cc04331a
Cho, B. (2009). Recent development and improvement for boron hydride-based catalytic asymmetric reduction of unsymmetrical ketones. Chemical Society Reviews, 38(2), 443-452. https://doi.org/10.1039/b811341f
Dohi, T. (2010). Recycling and catalytic approaches for the development of a rare-metal-free synthetic method using hypervalent iodine reagent. Chemical and Pharmaceutical Bulletin, 58(2), 135-142. https://doi.org/10.1248/cpb.58.135
Fujii, K., Todani, H., Ito, S., & Mikami, K. (2019). Design of phosphinic acid catalysts with the closest stereogenicity at the α-position: synthesis and application of α-stereogenic perfluoroalkyl phosphinic acid catalysts. Organic Letters, 21(9), 3387-3391. https://doi.org/10.1021/acs.orglett.9b01131
Huo, H., Shen, X., Wang, C., Zhang, L., Röse, P., Chen, L., … & Meggers, E. (2014). Asymmetric photoredox transition-metal catalysis activated by visible light. Nature, 515(7525), 100-103. https://doi.org/10.1038/nature13892
Huo, H., Shen, X., Wang, C., Zhang, L., Röse, P., Chen, L., … & Meggers, E. (2014). Asymmetric photoredox transition-metal catalysis activated by visible light. Nature, 515(7525), 100-103. https://doi.org/10.1038/nature13892
Ito, M., Kitahara, S., & Ikariya, T. (2005). Cp*ru(pn) complex-catalyzed isomerization of allylic alcohols and its application to the asymmetric synthesis of muscone. Journal of the American Chemical Society, 127(17), 6172-6173. https://doi.org/10.1021/ja050770g
Ji, C., Xiao, J., & Zeng, X. (2021). Recent progress in the stereoselective synthesis of (−)‐α‐kainic acid. Chemistryselect, 6(40), 10898-10909. https://doi.org/10.1002/slct.202102562
Li, B., Liú, D., Hu, Y., Chen, J., Zhang, Z., & Zhang, W. (2021). Nickel‐catalyzed asymmetric hydrogenation of hydrazones. European Journal of Organic Chemistry, 2021(23), 3421-3425. https://doi.org/10.1002/ejoc.202100642
Li, B., Liú, D., Hu, Y., Chen, J., Zhang, Z., & Zhang, W. (2021). Nickel‐catalyzed asymmetric hydrogenation of hydrazones. European Journal of Organic Chemistry, 2021(23), 3421-3425. https://doi.org/10.1002/ejoc.202100642
Li, Z., Wang, W., Song, J., Wang, R., Shi, J., Chi, Q., … & Pan, W. (2021). Copper-catalyzed cyclization/dimerization of tryptamines with o2/air as the sole oxidant: direct access to complex bispyrrolidino[2,3-b]indoline. The Journal of Organic Chemistry, 86(23), 17164-17172. https://doi.org/10.1021/acs.joc.1c02277
Page, P., Buckley, B., Farah, M., & Blacker, A. (2009). Binaphthalene‐derived iminium salt catalysts for highly enantioselective asymmetric epoxidation. European Journal of Organic Chemistry, 2009(20), 3413-3426. https://doi.org/10.1002/ejoc.200900252
Palo‐Nieto, C., Afewerki, S., Anderson, M., Tai, C., Berglund, P., & Córdova, A. (2016). Integrated heterogeneous metal/enzymatic multiple relay catalysis for eco-friendly and asymmetric synthesis. Acs Catalysis, 6(6), 3932-3940. https://doi.org/10.1021/acscatal.6b01031
Sawada, D., Kanai, a., & Shibasaki, M. (2000). Enantioselective total synthesis of epothilones a and b using multifunctional asymmetric catalysis. Journal of the American Chemical Society, 122(43), 10521-10532. https://doi.org/10.1021/ja002024b
Shibasaki, M., Kanai, M., Matsunaga, S., & Kumagai, N. (2009). Recent progress in asymmetric bifunctional catalysis using multimetallic systems. Accounts of Chemical Research, 42(8), 1117-1127. https://doi.org/10.1021/ar9000108
Wang, S., Chen, X., Bao, L., Liu, K., Bi, Y., Xue, Y., … & Zhang, Y. (2023). A magnetic fe3o4/modified bentonite composite as recyclable heterogeneous catalyst for synthesizing 2‐substituted benzimidazoles. Chemistryselect, 8(13). https://doi.org/10.1002/slct.202204930
Zhao, W. (2023). An asymmetric hydrogenation/n‐alkylation sequence for a step‐economical route to indolizidines and quinolizidines. Angewandte Chemie, 62(41). https://doi.org/10.1002/anie.202308836
Zhou, M., Dong, D., Zhu, B., Geng, H., Wang, Y., & Zhang, X. (2013). Rhodium-catalyzed enantioselective hydrogenation of β-acylamino nitroolefins: a new approach to chiral β-amino nitroalkanes. Organic Letters, 15(21), 5524-5527. https://doi.org/10.1021/ol4026843